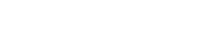
What If the Cosmological Constant Is Not Constant?
Season 10 Episode 23 | 15m 14sVideo has Closed Captions
Is Dark Energy Getting Weaker?
We know that the universe is getting bigger. And we know that the speed that the universe is getting bigger is also getting bigger. The standard assumption is that the acceleration rate is itself constant, which will result in ultimate heat death. But a recent survey of primordial sound waves frozen into the way galaxies are sprinkled through the universe reveals that this fate is now in question.
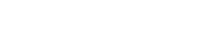
What If the Cosmological Constant Is Not Constant?
Season 10 Episode 23 | 15m 14sVideo has Closed Captions
We know that the universe is getting bigger. And we know that the speed that the universe is getting bigger is also getting bigger. The standard assumption is that the acceleration rate is itself constant, which will result in ultimate heat death. But a recent survey of primordial sound waves frozen into the way galaxies are sprinkled through the universe reveals that this fate is now in question.
How to Watch PBS Space Time
PBS Space Time is available to stream on pbs.org and the free PBS App, available on iPhone, Apple TV, Android TV, Android smartphones, Amazon Fire TV, Amazon Fire Tablet, Roku, Samsung Smart TV, and Vizio.
Providing Support for PBS.org
Learn Moreabout PBS online sponsorshipWe know that the universe is getting bigger.
And we know that the speed that the universe is getting bigger is also getting bigger.
The standard assumption is that the cosmic acceleration rate is itself is at least constant, which will surely result in ultimate heat death of the universe.
But a recent survey of primordial sound waves frozen into the way galaxies are sprinkled through the universe reveals that this fate is now in question.
Back in 1998 we discovered that the expansion of the universe is accelerating.
This rates as one of the most important findings in the past several decades in physics.
That’s because dark energy—our placeholder name for whatever causes this acceleration—is utterly mysterious and may be the clue we need to move beyond our current understanding of physics.
To be clear, we do understand the math of how dark energy works—just add a constant energy density to the fabric of space and you get accelerating expansion, represented by the cosmological constant in Einstein’s general theory of relativity.
These days, almost all of cosmology assumes something called the Lambda-CDM model—CDM for cold dark matter, not the topic of this video, and Lambda for the cosmological constant.
We even have a prime candidate for this sort of unchanging dark energy.
We expect fluctuations in the quantum fields to produce such a constant energy density, resulting in an “energy of the vacuum” that behaves exactly like a cosmological constant.
Problem is, with our current understanding of the quantum fields in our universe, we would expect those quantum fluctuations to most naturally do one of two things: to cancel out to exactly zero, or pile up to an enormous, universe-destroying value.
It’s very, very difficult to see how those fluctuations should produce such a tiny but non-zero vacuum energy without extreme fine-tuning of the field parameters.
There are proposals for how this fine-tuning may have happened—for example, we could be an extremely rare universe with a habitable value for the vacuum energy among a multitude of desolate ones.
But if you don’t like these anthropic arguments, we could also reject the idea that dark energy is vacuum energy at all.
For example it might be an undiscovered quantum field.
One thing that a new quantum field might be expected to do is to change in strength over time, which would lead to a changing rate of acceleration.
So how would we look for a changing acceleration rate?
Detecting that the expansion was accelerating at all required us to map the changing size of the universe back in time.
This was done by determining the distances to a type of supernova—type 1a supernovae, or exploding white dwarf stars.
This approach gave us the expansion history over the most recent half of the age of the universe.
That’s enough to show the expansion rate is changing, but not enough to see whether the change in that rate is itself changing.
We also have a data point at the beginning of time with the cosmic microwave background radiation.
If you compare the expansion rates predicted by these two methods assuming a constant acceleration, you get a mismatch.
The CMB measure gives an expansion rate smaller than the supernova measure.
This is the now-famous crisis in cosmology.
Many believe that there’s a systematic error in these measurements somewhere.
But another possibility is that the assumption of constant acceleration is wrong.
It’s starting to sound like it would be a good idea to properly map the expansion history back through as much of cosmic time as possible with a single method.
Then the acceleration history should be clear, and with it the constancy or non-constancy of dark energy.
Unfortunately we can’t see supernovae at large enough distances, and the CMB is only at the furthest distance by definition.
But we do have another option: Baryon Acoustic Oscillations—BAOs.
BAOs are like standard rulers that stretch all the way back to the beginning of the universe.
We talked about them before, but let me give you a refresher.
In the few hundred thousand years right after the big bang, the entire universe was filled with hot plasma and no stars or even atoms had formed.
This plasma flowed into the regions of higher gravity that would later collapse into clusters of galaxies.
As these massive inflows crashed together, vast sound waves reverberated back out, creating expanding shells of higher density material.
These sound waves could travel because matter was locked together with the intense radiation that filled the universe.
But as the universe also expanded and cooled, and at some point the first atoms formed; matter and light were no longer locked together and so our sound waves stopped moving.
The pattern of those waves became frozen into the distribution of matter at that moment a few hundred thousand years after the big bang.
Later, galaxies and clusters would form from this matter.
Most galaxies would form near the centers of large overdensities—but there should be much weaker shells of overdensities surrounding each of these where the sound waves were frozen.
We see these baryon acoustic oscillations in the cosmic microwave background—the light carried to us from the moment of freezing.
But we also see that there are rings of galaxies distantly surrounding galaxy clusters.
This is an incredibly faint signal—observable only as a slightly higher probability of finding galaxies separated by the diameter that these rings have today.
That diameter is called the sound horizon of the BAOs—the maximum distance sound travelled before freezing—and it’s around 500 million light years after having expanded with the rest of the universe.
This number is our standard ruler.
We know how big the sound horizon should be at any point in the history of the expanding universe.
By measuring the angular size of this horizon on the sky, we can find the true physical distance corresponding to the galaxies on these BAO rings.
With one more ingredient, that translates to an expansion history of the universe.
That final ingredient is redshift, and this is exactly what the Dark Energy Spectroscopic Instrument measures.
DESI is a pretty amazing instrument and survey.
It measures redshifts—the amount that light is stretched as it travels to us through an expanding universe.
We’ve been able to measure redshifts since the 20’s—that’s how we discovered the expansion of the universe in the first place.
But DESI is able to measure redshifts of 5000 galaxies or quasars at a time.
For every patch of the sky, 5000 robots place as many optical fibers at precise positions on a plate, corresponding to the precise locations of galaxies in that patch.
Light from those galaxies travels the optical fiber down to a spectrograph, where it’s split into component wavelengths.
Characteristic spikes in emission due to known elements in each galaxy then reveal the redshifts.
In this way, DESI has been able to measure redshifts for 26 million galaxies and quasars so far, and the plan is to get to 40 million by the end of the survey.
This will ultimately result in a giant three dimensional map of the nearby universe over the past 11 billion years.
Combined with the size of the BAO rings at each redshift, this also gives us a map of the expansion history.
Back in April of this year, the DESI collaboration published a series of papers with the results from the first year of the survey—from those 6 million redshifts.
One of those papers covered the baryon acoustic oscillations, in which they determine constraints on a few cosmological parameters.
One of these is something called omega, which gives the so-called “equation of state” of dark energy.
It tells us how much pressure is being produced for a given energy density.
In Lambda CDM, we expect omega to equal exactly -1 for all of time, and the DESI results are in very good agreement with omega = -1 and constant dark energy, just as we have always assumed.
That sounds anticlimactic, but then something odd turned up.
The DESI team combined the sound horizon measurements from their redshift survey with sound horizon measurements from the two other types of survey: from the Planck satellite measurements of the cosmic microwave background at the beginning of time, and with a few supernova surveys of the more modern universe.
Adding these other surveys led to an unexpected result.
The combined data were better fit with a model with omega -1 and in which dark energy is getting weaker over time.
And that’s the case whether the CMB or supernova results were added to DESI separately or together.
The statistical significance of this result depends on which combination of datasets you look at.
It’s 2.6-sigma for DESI + CMB, and up to nearly 4-sigma with a particular one of the supernova samples included.
2.6-sigma is considered not statistically significant, although still worthy of curiosity and more observations.
4-sigma is getting exciting, even if it’s short of the normal 5-sigma threshold for a very likely positive detection.
But we should also be quite wary of that 4-sigma result because it depends on inferring distances from supernovae.
The BAO sound horizons measured this way are probably more subject to systematic errors than are the CMB results.
That’s because supernova distances depend on a longer chain of distance measurements, and so there are more places that some error could have crept in.
OK, let’s allow ourselves to get excited just for a minute and ask what this means if it’s true.
One of the more general models for non-constant dark energy is called “quintessence”, which has an equation of state that can change over time.
The DESI paper focuses on a version of this stuff called thawing quintessence, in which an initially constant dark energy sort of defrosts, becoming variable over time.
And Desi finds this model is more consistent with the various combined surveys than constant dark energy.
If dark energy behaves this way then it’s less likely that it will reverse course and start to get stronger in the future.
That may preclude the Big Rip scenario, in which dark energy gets so powerful that it shreds the fabric of space time.
So, the universe hopefully doesn’t end as we described in this episode.
The opposite extreme to the big rip is the big crunch, in which the expansion eventually slows, then turns around and the universe collapses into a reverse big bang—potentially even kickstarting a new universe.
We don’t expect that to happen even with no dark energy whatsoever.
The current expansion rate is enough to keep the universe expanding forever, even ignoring dark energy and including the slow-down from the inward gravitational pull of all galaxies.
If the density of dark energy is diminishing and eventually hits zero, that won’t stop eternal expansion—although it will hold off the accompanying heat death for a while longer.
To get a big crunch, dark energy would have to change in a more fundamental way—it would have to shift to a positive gravitational force rather than a negative one.
And that’s not impossible.
If the equation of state parameter omega also changes, and in particular rises from the current -1 to -⅓ or higher then dark energy stops behaving like dark energy at all and could work to recollapse the universe.
Then we might get a proper crunchy end to the universe after all.
But perhaps the most exciting outcome of discovering a non-constant dark energy is that it may constrain other physics.
We’ve been a bit stuck this past few decades, unable to move beyond general relativity and the standard model of particle physics.
Things like string theory seemed promising, but have trouble generating testable predictions.
String theory is especially challenging because it predicts a myriad of possible universes called the string landscape and it seems impossible to determine how to identify our universe in this space.
Well, in 2018 a team of theoretical physicists showed that only a subset of the string landscape should lead to stable universes, and that a requirement of that stability of that subset is that dark energy should diminish over time.
So perhaps we have our first tested prediction of string theory and string theorists are going to be pretty excited if the DESI result pans out.
OK, one final word.
I mentioned the crisis in cosmology—the tension between the CMB versus supernova measurements of the expansion rate as measured by the Hubble constant.
This Hubble tension could be resolved with a changing acceleration rate.
Unfortunately, the acceleration rate would have to increase to resolve the tension, not decrease as DESI is suggesting.
In fact the DESI results do also infer an expansion rate the hubble constant—and they actually support the Planck CMB result and not the supernova result.
So it seems we have to be tense about the Hubble constant for a while longer.
And once again, please remember the this result has not yet reached the threshold of statistical significance that we need for a confident declaration of a discovery.
We’ll learn more soon though.
DESI has actually completed its second year of observations, and its results will come out faster than the first year results, so we’ll see if the finding firms up or evaporates.
And in a few years, when DESI has observed all 40 million galaxies and quasars, and we have results from other current or future programs such as the Dark Energy Survey and the Vera Rubin observatory, we will gradually be able to hone in on a more precise value of the equation of state of dark energy.
And then we should know one way or another—do we have a cosmological constant or a cosmological variable.
And perhaps we’ll finally understand what dark energy actually is, and have evidence for the underlying theory of everything that supports this, and even know the final fate of our expanding space time.