An in vivo genetic screen for genes involved in spliced leader trans-splicing indicates a crucial role for continuous de novo spliced leader RNP assembly
- PMID: 28582530
- PMCID: PMC5737717
- DOI: 10.1093/nar/gkx500
An in vivo genetic screen for genes involved in spliced leader trans-splicing indicates a crucial role for continuous de novo spliced leader RNP assembly
Abstract
Spliced leader (SL) trans-splicing is a critical element of gene expression in a number of eukaryotic groups. This process is arguably best understood in nematodes, where biochemical and molecular studies in Caenorhabditis elegans and Ascaris suum have identified key steps and factors involved. Despite this, the precise details of SL trans-splicing have yet to be elucidated. In part, this is because the systematic identification of the molecules involved has not previously been possible due to the lack of a specific phenotype associated with defects in this process. We present here a novel GFP-based reporter assay that can monitor SL1 trans-splicing in living C. elegans. Using this assay, we have identified mutants in sna-1 that are defective in SL trans-splicing, and demonstrate that reducing function of SNA-1, SNA-2 and SUT-1, proteins that associate with SL1 RNA and related SmY RNAs, impairs SL trans-splicing. We further demonstrate that the Sm proteins and pICln, SMN and Gemin5, which are involved in small nuclear ribonucleoprotein assembly, have an important role in SL trans-splicing. Taken together these results provide the first in vivo evidence for proteins involved in SL trans-splicing, and indicate that continuous replacement of SL ribonucleoproteins consumed during trans-splicing reactions is essential for effective trans-splicing.
© The Author(s) 2017. Published by Oxford University Press on behalf of Nucleic Acids Research.
Figures
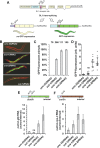
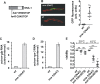
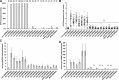
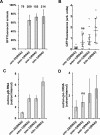
Similar articles
-
A nematode-specific ribonucleoprotein complex mediates interactions between the major nematode spliced leader snRNP and its target pre-mRNAs.Nucleic Acids Res. 2024 Jul 8;52(12):7245-7260. doi: 10.1093/nar/gkae321. Nucleic Acids Res. 2024. PMID: 38676950 Free PMC article.
-
A novel, essential trans-splicing protein connects the nematode SL1 snRNP to the CBC-ARS2 complex.Nucleic Acids Res. 2022 Jul 22;50(13):7591-7607. doi: 10.1093/nar/gkac534. Nucleic Acids Res. 2022. PMID: 35736244 Free PMC article.
-
New components of the spliced leader RNP required for nematode trans-splicing.Nature. 2002 Jun 6;417(6889):667-70. doi: 10.1038/nature00783. Nature. 2002. PMID: 12050671
-
trans-splicing: an update.Mol Biochem Parasitol. 1995 Jul;73(1-2):1-6. doi: 10.1016/0166-6851(94)00107-x. Mol Biochem Parasitol. 1995. PMID: 8577317 Review.
-
On the Possibility of an Early Evolutionary Origin for the Spliced Leader Trans-Splicing.J Mol Evol. 2017 Aug;85(1-2):37-45. doi: 10.1007/s00239-017-9803-y. Epub 2017 Jul 25. J Mol Evol. 2017. PMID: 28744787 Review.
Cited by
-
Automated profiling of gene function during embryonic development.Cell. 2024 Jun 6;187(12):3141-3160.e23. doi: 10.1016/j.cell.2024.04.012. Epub 2024 May 16. Cell. 2024. PMID: 38759650
-
A nematode-specific ribonucleoprotein complex mediates interactions between the major nematode spliced leader snRNP and its target pre-mRNAs.Nucleic Acids Res. 2024 Jul 8;52(12):7245-7260. doi: 10.1093/nar/gkae321. Nucleic Acids Res. 2024. PMID: 38676950 Free PMC article.
-
Gemin5-dependent RNA association with polysomes enables selective translation of ribosomal and histone mRNAs.Cell Mol Life Sci. 2022 Aug 20;79(9):490. doi: 10.1007/s00018-022-04519-4. Cell Mol Life Sci. 2022. PMID: 35987821 Free PMC article.
-
A novel, essential trans-splicing protein connects the nematode SL1 snRNP to the CBC-ARS2 complex.Nucleic Acids Res. 2022 Jul 22;50(13):7591-7607. doi: 10.1093/nar/gkac534. Nucleic Acids Res. 2022. PMID: 35736244 Free PMC article.
-
Functional and structural deficiencies of Gemin5 variants associated with neurological disorders.Life Sci Alliance. 2022 Apr 7;5(7):e202201403. doi: 10.26508/lsa.202201403. Print 2022 Jul. Life Sci Alliance. 2022. PMID: 35393353 Free PMC article.
References
-
- Pettitt J., Harrison N., Stansfield I., Connolly B., Müller B.. The evolution of spliced leader trans-splicing in nematodes. Biochem. Soc. Trans. 2010; 38:1125–1130. - PubMed
-
- Lasda E.L., Blumenthal T.. Trans-splicing. Wiley Interdiscip. Rev. RNA. 2011; 2:417–434. - PubMed
-
- Blumenthal T. Trans-Splicing and Operons in C. elegans. WormBook. 2012; The C. elegans Research Community. - PubMed
-
- Blumenthal T., Evans D., Link C.D., Guffanti A., Lawson D., Thierry-Mieg J., Thierry-Mieg D., Chiu W.L., Duke K., Kiraly M. et al. . A global analysis of Caenorhabditis elegans operons. Nature. 2002; 417:851–854. - PubMed
MeSH terms
Substances
Grants and funding
LinkOut - more resources
Full Text Sources
Other Literature Sources
Research Materials