3D architecture and a bicellular mechanism of touch detection in mechanosensory corpuscle
- PMID: 37703368
- PMCID: PMC10499330
- DOI: 10.1126/sciadv.adi4147
3D architecture and a bicellular mechanism of touch detection in mechanosensory corpuscle
Abstract
Mechanosensory corpuscles detect transient touch and vibration in the skin of vertebrates, enabling precise sensation of the physical environment. The corpuscle contains a mechanoreceptor afferent surrounded by lamellar cells (LCs), but corpuscular ultrastructure and the role of LCs in touch detection are unknown. We report the three-dimensional architecture of the avian Meissner (Grandry) corpuscle acquired using enhanced focused ion beam scanning electron microscopy and machine learning-based segmentation. The corpuscle comprises a stack of LCs interdigitated with terminal endings from two afferents. Simultaneous electrophysiological recordings from both cell types revealed that mechanosensitive LCs use calcium influx to trigger action potentials in the afferent and thus serve as physiological touch sensors in the skin. The elaborate architecture and bicellular sensory mechanism in the corpuscles, which comprises the afferents and LCs, create the capacity for nuanced encoding of the submodalities of touch.
Figures
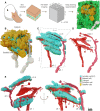
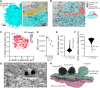
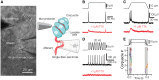
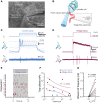
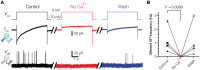
Update of
-
3D architecture and a bi-cellular mechanism of touch detection in mechanosensory corpuscle.bioRxiv [Preprint]. 2023 Apr 6:2023.04.05.535701. doi: 10.1101/2023.04.05.535701. bioRxiv. 2023. Update in: Sci Adv. 2023 Sep 15;9(37):eadi4147. doi: 10.1126/sciadv.adi4147. PMID: 37066170 Free PMC article. Updated. Preprint.
Similar articles
-
3D architecture and a bi-cellular mechanism of touch detection in mechanosensory corpuscle.bioRxiv [Preprint]. 2023 Apr 6:2023.04.05.535701. doi: 10.1101/2023.04.05.535701. bioRxiv. 2023. Update in: Sci Adv. 2023 Sep 15;9(37):eadi4147. doi: 10.1126/sciadv.adi4147. PMID: 37066170 Free PMC article. Updated. Preprint.
-
Mechanotransduction events at the physiological site of touch detection.Elife. 2023 Jan 6;12:e84179. doi: 10.7554/eLife.84179. Elife. 2023. PMID: 36607222 Free PMC article.
-
Lamellar cells in Pacinian and Meissner corpuscles are touch sensors.Sci Adv. 2020 Dec 16;6(51):eabe6393. doi: 10.1126/sciadv.abe6393. Print 2020 Dec. Sci Adv. 2020. PMID: 33328243 Free PMC article.
-
Tactile sensation in birds: Physiological insights from avian mechanoreceptors.Curr Opin Neurobiol. 2022 Jun;74:102548. doi: 10.1016/j.conb.2022.102548. Epub 2022 Apr 27. Curr Opin Neurobiol. 2022. PMID: 35489134 Free PMC article. Review.
-
Peripheral Mechanobiology of Touch-Studies on Vertebrate Cutaneous Sensory Corpuscles.Int J Mol Sci. 2020 Aug 27;21(17):6221. doi: 10.3390/ijms21176221. Int J Mol Sci. 2020. PMID: 32867400 Free PMC article. Review.
Cited by
-
Structural and functional dissection of the Pacinian corpuscle reveals an active role of the inner core in touch detection.bioRxiv [Preprint]. 2024 Aug 26:2024.08.24.609509. doi: 10.1101/2024.08.24.609509. bioRxiv. 2024. PMID: 39253434 Free PMC article. Preprint.
-
A helping hand: roles for accessory cells in the sense of touch across species.Front Cell Neurosci. 2024 Feb 16;18:1367476. doi: 10.3389/fncel.2024.1367476. eCollection 2024. Front Cell Neurosci. 2024. PMID: 38433863 Free PMC article. Review.
-
A Hierarchical Mechanotransduction System: From Macro to Micro.Adv Sci (Weinh). 2024 Mar;11(11):e2302327. doi: 10.1002/advs.202302327. Epub 2023 Dec 25. Adv Sci (Weinh). 2024. PMID: 38145330 Free PMC article. Review.
References
-
- S. S. Ranade, S. H. Woo, A. E. Dubin, R. A. Moshourab, C. Wetzel, M. Petrus, J. Mathur, V. Bégay, B. Coste, J. Mainquist, A. J. Wilson, A. G. Francisco, K. Reddy, Z. Qiu, J. N. Wood, G. R. Lewin, A. Patapoutian, Piezo2 is the major transducer of mechanical forces for touch sensation in mice. Nature 516, 121–125 (2014). - PMC - PubMed
MeSH terms
Substances
Grants and funding
LinkOut - more resources
Full Text Sources