Elasmobranch genome sequencing reveals evolutionary trends of vertebrate karyotype organization
- PMID: 37591668
- PMCID: PMC10620051
- DOI: 10.1101/gr.276840.122
Elasmobranch genome sequencing reveals evolutionary trends of vertebrate karyotype organization
Abstract
Genomic studies of vertebrate chromosome evolution have long been hindered by the scarcity of chromosome-scale DNA sequences of some key taxa. One of those limiting taxa has been the elasmobranchs (sharks and rays), which harbor species often with numerous chromosomes and enlarged genomes. Here, we report the chromosome-scale genome assembly for the zebra shark Stegostoma tigrinum, an endangered species that has a relatively small genome among sharks (3.71 Gb), as well as for the whale shark Rhincodon typus Our analysis using a male-female comparison identified an X Chromosome, the first genomically characterized shark sex chromosome. The X Chromosome harbors the Hox C cluster whose intact linkage has not been shown for an elasmobranch fish. The sequenced shark genomes show a gradualism of chromosome length with remarkable length-dependent characteristics-shorter chromosomes tend to have higher GC content, gene density, synonymous substitution rate, and simple tandem repeat content as well as smaller gene length and lower interspersed repeat content. We challenge the traditional binary classification of karyotypes as with and without so-called microchromosomes. Even without microchromosomes, the length-dependent characteristics persist widely in nonmammalian vertebrates. Our investigation of elasmobranch karyotypes underpins their unique characteristics and provides clues for understanding how vertebrate karyotypes accommodate intragenomic heterogeneity to realize a complex readout. It also paves the way to dissecting more genomes with variable sizes to be sequenced at high quality.
© 2023 Yamaguchi et al.; Published by Cold Spring Harbor Laboratory Press.
Figures
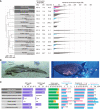
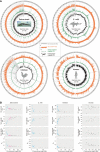
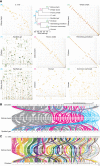
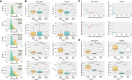
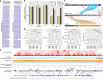
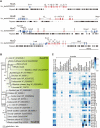
Similar articles
-
Cell culture-based karyotyping of orectolobiform sharks for chromosome-scale genome analysis.Commun Biol. 2020 Nov 6;3(1):652. doi: 10.1038/s42003-020-01373-7. Commun Biol. 2020. PMID: 33159152 Free PMC article.
-
Shark genomes provide insights into elasmobranch evolution and the origin of vertebrates.Nat Ecol Evol. 2018 Nov;2(11):1761-1771. doi: 10.1038/s41559-018-0673-5. Epub 2018 Oct 8. Nat Ecol Evol. 2018. PMID: 30297745
-
Draft sequencing and assembly of the genome of the world's largest fish, the whale shark: Rhincodon typus Smith 1828.BMC Genomics. 2017 Jul 14;18(1):532. doi: 10.1186/s12864-017-3926-9. BMC Genomics. 2017. PMID: 28709399 Free PMC article.
-
Why Do Some Vertebrates Have Microchromosomes?Cells. 2021 Aug 24;10(9):2182. doi: 10.3390/cells10092182. Cells. 2021. PMID: 34571831 Free PMC article. Review.
-
Hox gene clusters of early vertebrates: do they serve as reliable markers for genome evolution?Genomics Proteomics Bioinformatics. 2011 Jun;9(3):97-103. doi: 10.1016/S1672-0229(11)60012-0. Genomics Proteomics Bioinformatics. 2011. PMID: 21802046 Free PMC article. Review.
Cited by
-
Understanding vertebrate immunity through comparative immunology.Nat Rev Immunol. 2025 Feb;25(2):141-152. doi: 10.1038/s41577-024-01083-9. Epub 2024 Sep 24. Nat Rev Immunol. 2025. PMID: 39317775 Review.
-
The complete mitogenome of the Atlantic longnose chimaera Rhinochimaera atlantica (Holt & Byrne, 1909).Mitochondrial DNA B Resour. 2024 Jul 17;9(7):886-891. doi: 10.1080/23802359.2024.2378127. eCollection 2024. Mitochondrial DNA B Resour. 2024. PMID: 39027115 Free PMC article.
-
Convergent gene losses and pseudogenizations in multiple lineages of stomachless fishes.Commun Biol. 2024 Apr 3;7(1):408. doi: 10.1038/s42003-024-06103-x. Commun Biol. 2024. PMID: 38570609 Free PMC article.
-
Comparative genomics illuminates karyotype and sex chromosome evolution of sharks.Cell Genom. 2024 Aug 14;4(8):100607. doi: 10.1016/j.xgen.2024.100607. Epub 2024 Jul 11. Cell Genom. 2024. PMID: 38996479 Free PMC article.
-
The evolution of tenascins.BMC Ecol Evol. 2024 Sep 14;24(1):121. doi: 10.1186/s12862-024-02306-2. BMC Ecol Evol. 2024. PMID: 39277743 Free PMC article.
References
Publication types
MeSH terms
LinkOut - more resources
Full Text Sources
Miscellaneous