Body fluid dynamics: back to the future
- PMID: 22034644
- PMCID: PMC4096826
- DOI: 10.1681/ASN.2011080865
Body fluid dynamics: back to the future
Abstract
Pioneering investigations conducted over a half century ago on tonicity, transcapillary fluid exchange, and the distribution of water and solute serve as a foundation for understanding the physiology of body fluid spaces. With passage of time, however, some of these concepts have lost their connectivity to more contemporary information. Here we examine the physical forces determining the compartmentalization of body fluid and its movement across capillary and cell membrane barriers, drawing particular attention to the interstitium operating as a dynamic interface for water and solute distribution rather than as a static reservoir. Newer work now supports an evolving model of body fluid dynamics that integrates exchangeable Na(+) stores and transcapillary dynamics with advances in interstitial matrix biology.
Figures
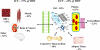
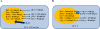
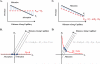
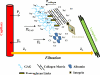
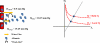
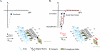
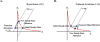
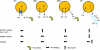
Similar articles
-
Microcirculation of the alimentary tract I. Physiology of transcapillary fluid and solute exchange.Gastroenterology. 1983 Apr;84(4):846-68. Gastroenterology. 1983. PMID: 6337911 Review. No abstract available.
-
Forces involved in transcapillary fluid movement in exercising cat skeletal muscle.Acta Physiol Scand. 1990 Oct;140(2):221-36. doi: 10.1111/j.1748-1716.1990.tb08994.x. Acta Physiol Scand. 1990. PMID: 2267951
-
Distribution of body fluids: local mechanisms guarding interstitial fluid volume.J Physiol (Paris). 1984;79(6):395-400. J Physiol (Paris). 1984. PMID: 6399307 Review.
-
Capillary Fluid Exchange: Regulation, Functions, and Pathology.San Rafael (CA): Morgan & Claypool Life Sciences; 2010. San Rafael (CA): Morgan & Claypool Life Sciences; 2010. PMID: 21452435 Free Books & Documents. Review.
-
Body fluid compartments.Anaesth Intensive Care. 1977 Nov;5(4):284-94. doi: 10.1177/0310057X7700500403. Anaesth Intensive Care. 1977. PMID: 596611
Cited by
-
Using noninvasive imaging to assess manual lymphatic drainage on lymphatic/venous responses in a spaceflight analog.NPJ Microgravity. 2024 Oct 3;10(1):93. doi: 10.1038/s41526-024-00429-w. NPJ Microgravity. 2024. PMID: 39362907 Free PMC article.
-
Relationship between Plasma and Saliva Urea Nitrogen Concentrations in New Zealand Red Deer Calves (Cervus elaphus).Animals (Basel). 2024 Sep 3;14(17):2565. doi: 10.3390/ani14172565. Animals (Basel). 2024. PMID: 39272350 Free PMC article.
-
Evolution of Bioelectric Membrane Potentials: Implications in Cancer Pathogenesis and Therapeutic Strategies.J Membr Biol. 2024 Aug 25. doi: 10.1007/s00232-024-00323-2. Online ahead of print. J Membr Biol. 2024. PMID: 39183198
-
Dysnatremia as a Mortality Marker in Intensive Care Patients with SARS-CoV-2 Infection: A Retrospective Study.Medicina (Kaunas). 2024 Jun 21;60(7):1019. doi: 10.3390/medicina60071019. Medicina (Kaunas). 2024. PMID: 39064448 Free PMC article.
-
Association between serum chloride and in-hospital mortality in congestive heart failure with diabetes: Data from the MIMIC-IV database.J Diabetes Metab Disord. 2023 Dec 15;23(1):859-870. doi: 10.1007/s40200-023-01362-7. eCollection 2024 Jun. J Diabetes Metab Disord. 2023. PMID: 38932886
References
-
- Fanestil DD. Compartmentation of Body Water. In: NARINS RG, editor. Clinical Disorders of Fluid and Electrolyte Metabolism. Fifth ed. McGraw-Hill; New York: 1994. pp. 3–20.
-
- Edelman IS, Leibman J. Anatomy of body water and electrolytes. Am J Med. 1959;27:256–77. - PubMed
-
- Rose BD, Post TW. Clinical Physiology of Acid-Base and Electrolyte Disorders. McGraw-Hill; New York: 2001.
-
- Moore FD, Olesen KH, McMurrey JD, Parker HV, Ball MR, Boyden CM. The Body Cell Mass and Its Supporting Environment. Saunders; Philadelphia, W.B.: 1963.
-
- Chumlea WC, Guo SS, Zeller CM, Reo NV, Baumgartner RN, Garry PJ, Wang J, Pierson RN, Jr., Heymsfield SB, Siervogel RM. Total body water reference values and prediction equations for adults. Kidney Int. 2001;59:2250–8. - PubMed
Publication types
MeSH terms
Grants and funding
LinkOut - more resources
Full Text Sources