Structure-based analysis of DNA sequence patterns guiding nucleosome positioning in vitro
- PMID: 20232936
- PMCID: PMC2993692
- DOI: 10.1080/073911010010524947
Structure-based analysis of DNA sequence patterns guiding nucleosome positioning in vitro
Abstract
Recent studies of genome-wide nucleosomal organization suggest that the DNA sequence is one of the major determinants of nucleosome positioning. Although the search for underlying patterns encoded in nucleosomal DNA has been going on for about 30 years, our knowledge of these patterns still remains limited. Based on our evaluations of DNA deformation energy, we developed new scoring functions to predict nucleosome positioning. There are three principal differences between our approach and earlier studies: (i) we assume that the length of nucleosomal DNA varies from 146 to 147 bp; (ii) we consider the anisotropic flexibility of pyrimidine-purine (YR) dimeric steps in the context of their neighbors (e.g., YYRR versus RYRY); (iii) we postulate that alternating AT-rich and GC-rich motifs reflect sequence-dependent interactions between histone arginines and DNA in the minor groove. Using these functions, we analyzed 20 nucleosome positions mapped in vitro at single nucleotide resolution (including clones 601, 603, 605, the pGUB plasmid, chicken beta-globin and three 5S rDNA genes). We predicted 15 of the 20 positions with 1-bp precision, and two positions with 2-bp precision. The predicted position of the '601' nucleosome (i.e., the optimum of the computed score) deviates from the experimentally determined unique position by no more than 1 bp - an accuracy exceeding that of earlier predictions. Our analysis reveals a clear heterogeneity of the nucleosomal sequences which can be divided into two groups based on the positioning 'rules' they follow. The sequences of one group are enriched by highly deformable YR/YYRR motifs at the minor-groove bending sites SHL+/- 3.5 and +/- 5.5, which is similar to the alpha-satellite sequence used in most crystallized nucleosomes. Apparently, the positioning of these nucleosomes is determined by the interactions between histones H2A/H2B and the terminal parts of nucleosomal DNA. In the other group (that includes the '601' clone) the same YR/YYRR motifs occur predominantly at the sites SHL +/- 1.5. The interaction between the H3/H4 tetramer and the central part of the nucleosomal DNA is likely to be responsible for the positioning of nucleosomes of this group, and the DNA trajectory in these nucleosomes may differ in detail from the published structures. Thus, from the stereochemical perspective, the in vitro nucleosomes studied here follow either an X-ray-like pattern (with strong deformations in the terminal parts of nucleosomal DNA), or an alternative pattern (with the deformations occurring predominantly in the central part of the nucleosomal DNA). The results presented here may be useful for genome-wide classification of nucleosomes, linking together structural and thermodynamic characteristics of nucleosomes with the underlying DNA sequence patterns guiding their positions.
Figures
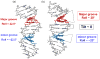
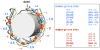
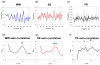
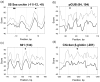
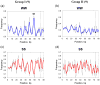
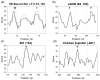
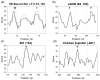
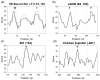
Similar articles
-
Sequence-dependent Kink-and-Slide deformations of nucleosomal DNA facilitated by histone arginines bound in the minor groove.J Biomol Struct Dyn. 2010 Jun;27(6):843-59. doi: 10.1080/07391102.2010.10508586. J Biomol Struct Dyn. 2010. PMID: 20232937 Free PMC article.
-
DNA architecture, deformability, and nucleosome positioning.J Biomol Struct Dyn. 2010 Jun;27(6):725-39. doi: 10.1080/073911010010524943. J Biomol Struct Dyn. 2010. PMID: 20232929 Free PMC article.
-
Nucleosomal locations of dominant DNA sequence motifs for histone-DNA interactions and nucleosome positioning.J Mol Biol. 2004 May 7;338(4):695-709. doi: 10.1016/j.jmb.2004.03.032. J Mol Biol. 2004. PMID: 15099738
-
Working the kinks out of nucleosomal DNA.Curr Opin Struct Biol. 2011 Jun;21(3):348-57. doi: 10.1016/j.sbi.2011.03.006. Epub 2011 Apr 7. Curr Opin Struct Biol. 2011. PMID: 21482100 Free PMC article. Review.
-
A structural perspective on the where, how, why, and what of nucleosome positioning.J Biomol Struct Dyn. 2010 Jun;27(6):803-20. doi: 10.1080/07391102.2010.10508585. J Biomol Struct Dyn. 2010. PMID: 20232935 Review.
Cited by
-
Comprehensive computational analysis of epigenetic descriptors affecting CRISPR-Cas9 off-target activity.BMC Genomics. 2022 Dec 6;23(1):805. doi: 10.1186/s12864-022-09012-7. BMC Genomics. 2022. PMID: 36474180 Free PMC article.
-
Sequence-dependent Kink-and-Slide deformations of nucleosomal DNA facilitated by histone arginines bound in the minor groove.J Biomol Struct Dyn. 2010 Jun;27(6):843-59. doi: 10.1080/07391102.2010.10508586. J Biomol Struct Dyn. 2010. PMID: 20232937 Free PMC article.
-
Training-free atomistic prediction of nucleosome occupancy.Proc Natl Acad Sci U S A. 2014 Apr 29;111(17):6293-8. doi: 10.1073/pnas.1404475111. Epub 2014 Apr 14. Proc Natl Acad Sci U S A. 2014. PMID: 24733939 Free PMC article.
-
Molecular mechanism of UV damage modulation in nucleosomes.Comput Struct Biotechnol J. 2022 Sep 14;20:5393-5400. doi: 10.1016/j.csbj.2022.08.071. eCollection 2022. Comput Struct Biotechnol J. 2022. PMID: 36212527 Free PMC article.
-
Genome wide nucleosome mapping for HSV-1 shows nucleosomes are deposited at preferred positions during lytic infection.PLoS One. 2015 Feb 24;10(2):e0117471. doi: 10.1371/journal.pone.0117471. eCollection 2015. PLoS One. 2015. PMID: 25710170 Free PMC article.
References
Publication types
MeSH terms
Substances
Grants and funding
LinkOut - more resources
Full Text Sources
Miscellaneous