ABOVE: © SCIENCE PHOTO LIBRARY, NATURE PICTURE LIBRARY, ALEX MUSTARD
It was the seventh night after the August full moon, the peak spawning window predicted for the mountainous star coral. We loaded the boat and headed out to a coral reef near Summerland Key, Florida, around sunset. As we donned our scuba gear and jumped in, the sun dipped below the horizon.
We’d been monitoring this site for three years now, watching and waiting for signs that the restored corals we’d planted in 2015 had achieved the next critical developmental milestone in their life cycle—sexual reproduction. In 2018, a storm prevented us from monitoring the corals; in 2019, the site was hit hard by the deadly stony coral tissue loss disease (SCTLD) outbreak, and we did not witness any spawning activity. Last year, however, a preliminary investigation provided reason for hope: we confirmed that the corals were sexually mature...
We began monitoring the corals around 9:30 PM that August night. We swam in continuous loops to check dozens of the hundreds of corals that in preceding years we had “outplanted,” placed onto the dying reef after raising them in our land-based spawning and nursery facility at Mote Marine Laboratory’s Elizabeth Moore International Center for Coral Reef Research & Restoration on Summerland Key. Specifically, we were looking to see if any of our outplanted mountainous star corals (Orbicella faveolata) had “set”—shifted the pink-orange bundles of eggs and sperm into the mouths of the polyps, where they are visible to the naked eye. Once we saw the gamete bundles in position for release, we’d know that spawning was imminent.
The colorful spheres hovered momentarily before gracefully floating upwards to the ocean surface in an ascent that resembled reverse snowfall.
The previous night, our team had observed that a single colony of this mounding coral species had set around 11:00 PM, so that became our target time for expecting setting in subsequent nights. On this night, when the hour came and went, we began to get nervous. We were frantically checking all outplants, over and over. Finally, at 11:05 PM, we heard the signal: RAP! RAP! RAP! Someone was smacking their dive light against their tank. We sped through the darkness and converged at the source of the sound. Mote biologist Sarah Hamlyn was hovering over a large coral head and excitedly pointing at three adjacent colonies clearly setting. We all hung there, motionless in the water, with eyes peeled and cameras in hand, for what seemed like an eternity. Then, at 11:15 PM, the polyps erupted, ejecting thousands of tiny pink gamete bundles into the water around us. The colorful spheres hovered momentarily before gracefully floating upwards to the ocean surface in an ascent that resembled reverse snowfall.
Just as quickly as it began, it ended; the whole show lasted less than a minute. We erupted into a quick burst of bubbled screams before peeling off to go check on the other outplants. That night, we observed nine different outplants across three different coral heads spawn within a 10-minute window. Given that there were only five divers with a short window of opportunity to observe spawning, and the fact that we have hundreds of outplants dispersed across numerous coral heads, it’s likely that these nine were but a few of the many restored corals that spawned that night. The dive culminated in more underwater cheers, hugs, and dancing in the celebratory, electric-blue glow of bioluminescent plankton.
Apart from being a significant institution-wide achievement for us, these observations provide hope for the future of coral reefs around the world. For several decades, researchers have documented dramatic declines in living coral cover across the globe. Florida’s Coral Reef, for example, has lost more than 90 percent of its corals since the 1960s, and Australia’s Great Barrier Reef has lost at least 50 percent of its corals just in the last few decades. Numerous issues including disease outbreaks, habitat degradation, and increasing ocean temperature and acidification are to blame. In most cases, there is little evidence of population recovery after major disturbance events. But restoring reefs by outplanting genetically diverse, stress-tolerant corals on a large scale has rarely been attempted. That night last August, we witnessed the first corals of any slow-growing, massive or mounding species to have produced gametes and spawned after being outplanted back to the wild. And thanks to our novel strategy of planting many little coral colonies in proximity on the same coral head, they did so in just five years, far less than the decades it may take a wild colony or a single outplant to mature.
Devastating as it is to witness 50- to 100-year-old corals dying from disease or bleaching, the conclusion that you can’t replace a 50-year-old coral in a decade may no longer be true for these massive, slow-growing, reef-building species, providing hope that coral restoration efforts can help save one of the world’s most threatened ecosystems.
Coral restoration comes to the fore
In 1995, founders of the US and International Coral Reef Initiatives highlighted implementation of local and national level coral reef restoration as a high priority to achieve global impacts for coral conservation. Nevertheless, the scientific community has been slow to take up the challenge, and researchers and conservationists continue to debate the efficacy of coral restoration. As recently as 2014, major scientific reports documenting the devastating decline in corals over the past 40 years rarely identified coral restoration as a tool for stemming coral reef decline. Although the US National Oceanic and Atmospheric Administration (NOAA) conducted three major coral reef repairs between 1997 and 2002 in response to large vessel groundings on coral reefs in the Florida Keys and Puerto Rico, the purpose of the repairs was to stabilize the reef structure and mitigate localized biological damage directly caused by the grounding, not to recover corals lost from decades of local and global threats.
Only in the past decade have researchers begun to develop science-based coral restoration strategies in earnest. Scientists and practitioners around the world have now reported more than 250 coral restoration case studies, involving hundreds of thousands of outplanted corals. The majority of projects occur in the US, but restoration efforts are now increasing in other parts of the world, including the Great Barrier Reef, in response to recent major coral mortality events.
Only in the past decade have researchers begun to develop science-based coral restoration strategies in earnest.
Reef-building corals come in all different shapes, colors, and sizes. These species display wide variation in growth rates, tolerance to thermal stress, and resistance or resilience to different diseases and pollutants, and many are threatened by anthropogenic disturbances, including rapid climate change. To date, most restoration efforts have focused on branching coral species that grow quickly, especially in a nursery setting, because they are relatively easy to propagate. This is done by repeatedly fragmenting the corals into pieces as a form of asexual reproduction to grow up many coral colonies. This process is similar to how one might propagate a plant in their home, where cuttings from one plant are used to create new individuals. Within a few months, branches of coral are snipped off the colony and transported to the reef, where they are secured by a degradable cable to masonry nails hammered into the dead reef or hard-bottom substrate. Coral fragments are attached to the reef in arrays of five that all come from the same parent colony (that is, they have the same genotype), and within a year or two, they grow and fuse into a large, reproductively viable adult colony. Arrays are situated on the reef so that adjacent clusters are different genotypes, to maximize the potential for cross-fertilization when sexual reproduction occurs.
However, restoring the backbone of a reef requires massive, slow-growing species such as the boulder and brain corals. These species are much harder to propagate for restoration because they typically grow only a few millimeters a year and usually need to be brought into the lab to be cut, whereas branching corals can be easily fragmented in the field. For the last several years, we have been propagating and outplanting these species using novel techniques that expedite their growth and maturation. We are now implementing these approaches on the reefs of the Florida Keys—and just in the nick of time. The reef system is currently experiencing the largest coral disease outbreak in reported history.
See “As Disease Batters Florida Reefs, Scientists and Community Fight Back”
How to quickly grow slow-growing coral
The mountainous star coral (O. faveolata) is native to the Caribbean and western Atlantic, and is a foundational species that helps build the backbone of Florida’s Coral Reef. Owing to severe declines in abundance over recent decades, it was listed as threatened under the Endangered Species Act in 2014. New coral offspring are failing to show up on reefs after annual reproductive events, leading to degraded, low-density populations that cannot sustain themselves via sexual reproduction. This has pushed Florida’s coral reefs into a state of functional extinction, no longer providing reef structure and critical habitat. When the devastating stony coral tissue loss disease (SCTLD), a contagious, waterborne disease caused by an unidentified pathogen, began spreading through the Keys in 2016, with mortality rates in excess of 90 percent for the most susceptible coral species, it added insult to injury.
Over the past 10 years, we have outplanted more than 100,000 fast- and slow-growing coral colonies, including some 26,000 in 2020, to reefs throughout the Keys. We are currently propagating 17 species, including O. faveolata, while actively incorporating diverse stress-tolerant coral genotypes to impart resilience to disease, ocean warming, and acidification. Although it’s possible that scientists could use genetic manipulation tools to design more-resilient corals than currently appear in nature, we focus on using genetic varieties that already exist within the endemic species of the region, with the aim of achieving sexually mature, self-sustaining, species-rich, and genetically diverse coral reefs as quickly as possible.
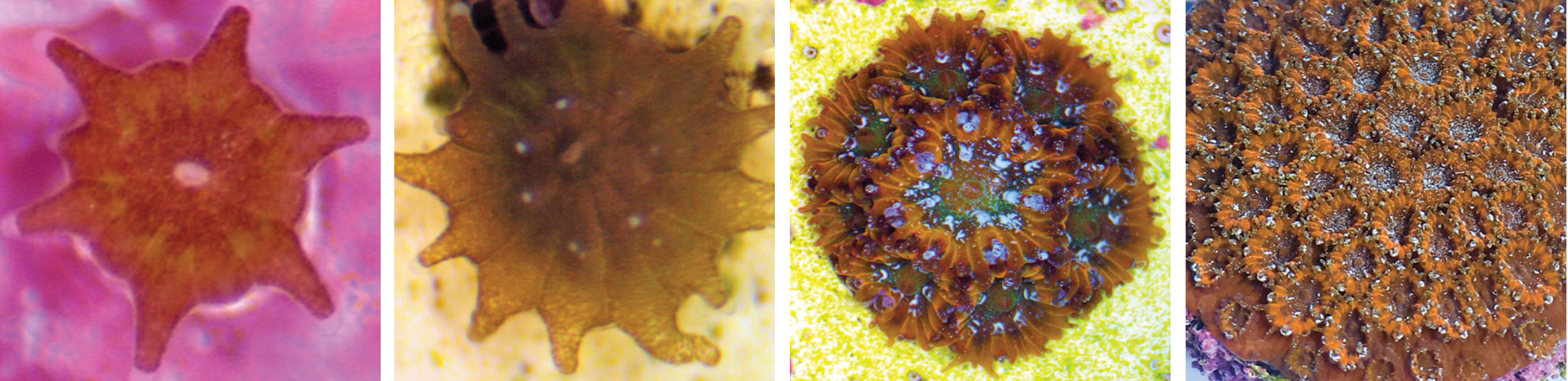
Importantly, in addition to large-scale asexual propagation, we incorporate new sexually produced genotypes of both branching and boulder coral species into our restoration pipeline each year. This approach ensures that our coral gene pool used for restoration remains diverse. Through assisted sexual reproduction efforts, we produce new generations of coral offspring each year, grow them to six months or one year old, depending on the species, and then use the asexual technique of microfragmentation to more rapidly increase the amount of coral tissue for each genotype.
While fragmentation with fast-growing branching corals is now commonplace, the field remains limited in its ability to regenerate massive, reef-building corals. To tackle this issue, we recently developed and began employing a new approach that combines microfragmentation of reef-building species and outplanting arrays of those microfragments onto dead coral heads. If employed systematically on a large scale, this strategy should accelerate reef recovery.
In 2013, we obtained colonies of mountainous star coral previously rescued by the Florida Keys National Marine Sanctuary from a construction site in Key West. On Mote’s Summerland Key campus, we fragmented the corals into pieces less than 1 cm in diameter and grew them for several months in our land-based nursery. Once the fragments reached around 3 cm, we outplanted them on patch reefs—isolated groupings of coral that are in close proximity to each other but are physically separated by sand rings—off Cook Island near Newfound Harbor in the Lower Keys. In 2014, we outplanted dozens of arrays comprising seven genetically identical fragments; from 2015 to 2017, we outplanted dozens more with 20 fragments in each array. Because fragments within an array are clonal, when they grow and eventually come in contact, they recognize one another as “self” and fuse together, essentially “reskinning” the dead coral skeleton with living healthy tissue. Between two and three years after outplanting, all arrays fully fused, creating whole coral colonies ranging from 15 cm to 30 cm in diameter.
This accelerated development breakthrough of up to 50 times natural growth rates is critical because the time required for corals to reach sexual maturity is size-dependent, not age-dependent; colonies of the mountainous star coral have to reach 10–30 cm before they are able to sexually reproduce. Normally, it would take decades for this species to grow to this size in the wild. When we saw our outplants spawn that memorable August night, it demonstrated that these fused colonies had indeed reached sexual maturity in record time.
The Microfragmentation ProcessAt Mote Marine Laboratory, our group has developed a new approach to restoring corals, depicted below. Already, we have seen new coral form across the dead skeleton of massive brain, boulder, star, and mounding coral structures in just one or two years, instead of the hundreds of years it might take a reef to regenerate on its own. Sexual reproduction is vital to the persistence of coral populations, but sexual maturity is size-dependent in reef corals, so expediting the growth of larger corals should support faster population and reef recovery. ![]() © JULIA MOORE, WWW.MOOREILLUSTRATIONS.COM When corals reproduce sexually in the wild, we collect bundles of their sperm and eggs, which we bring back to the lab and use to produce new baby corals. | |
![]() © JULIA MOORE, WWW.MOOREILLUSTRATIONS.COM | We also breed nursery-raised corals to produce new, genetically diverse, stress-tolerant offspring. |
![]() © JULIA MOORE, WWW.MOOREILLUSTRATIONS.COM | We generate large numbers of corals asexually by microfragmenting the colonies to produce clones. |
![]() © JULIA MOORE, WWW.MOOREILLUSTRATIONS.COM | We test coral genetic varieties for resilience to disease, climate change, and related stressors. |
![]() © JULIA MOORE, WWW.MOOREILLUSTRATIONS.COM | We plant coral fragments representing different genetic varieties and species onto damaged reefs to support resilience-based, multi-species restoration. |
![]() © JULIA MOORE, WWW.MOOREILLUSTRATIONS.COM | We monitor the outplanted corals as they grow, fuse together, and reach sexual maturity. |
The importance of sexual reproduction
Across the animal kingdom, sexual reproduction is a source of genetic variation that is critical for population survival and long-term persistence, and corals are no exception. For restored coral populations to survive long into the future, they will need sex to withstand rapid environmental change and accelerate adaptive evolution. To achieve sexually reproducing coral populations is a challenge, not only because slow-growing species take a long time to reach sexual maturity, but because once they do, reproduction is a relatively rare event. Most reef-building corals are hermaphrodites, producing both male and female gametes, which are broadcast into the water column for external fertilization with gametes of nearby corals of the same species. Such mass synchronized spawning events typically occur just once a year, and acute stressors such as bleaching events, hurricanes, and disease outbreaks can arrest gamete development and prevent spawning altogether.
Last summer, we confirmed sexual maturity just days before the corals’ expected 2020 spawning window, which typically occurs following the first full moon in August. Even before witnessing the spawning event that exciting August night, we knew that the corals were producing gametes. At the end of July, we took small core samples from a subset of outplants looking for the tell-tale pink-orange or “coral” color of the eggs. With the first few samples, we were unsure whether gametes were present, so we placed the cores in tubes to take back to the lab for further inspection. Then, on the second to last core, we found what we were looking for: a bright pink-orange spot. We used an underwater camera to take a macro shot and zoomed in on the image. There, without a doubt, was a string of mature eggs. Next to them—less obvious, but still visible—was a ribbon of sperm. These massive corals were ready to become parents in the wild. A true cause for celebration!
When the time came in August, not only did the corals in our study spawn during the predicted peak spawning window, but they also spawned with high synchrony. Spawning synchrony is critical to fertilization success, and having predictable spawning timing, both in terms of days after the full moon and minutes after sunset, means these outplants have developed reproductive rhythms consistent with wild colonies. Hence, they are capable of breeding with one another and with wild colonies, which should help to increase genetic diversity of coral offspring.
Witnessing our restored corals spawn was even more gratifying because these outplants survived a bleaching event in 2015, a Category 4 hurricane (Irma) in 2017, and the 2019 outbreak of SCTLD. In fact, two of the outplants we saw spawn had multiple SCTLD lesions in the spring of 2019, were treated with antibiotics (administered in a custom medium via syringe on the border of disease lesions), and recovered. This demonstrates that combining acute interventions such as antibiotics, which can help in the short term to prevent complete mortality, with resilience-based restoration strategies will work over the long term to help corals survive stressful environmental conditions.
Only a handful of published cases of coral reef restoration projects document corals reaching sexual maturity, and all previous cases pertained to fast-growing corals known as acroporids (genus Acropora). This was the first documentation of a slow-growing, massive coral species spawning, and it did so in a similar timeframe as did outplanted acroporids.
Once the outplants reach sexual maturity, their value extends beyond their ability to breed in the wild; the outplants can serve as a source of gametes for assisted sexual reproduction projects as part of continued research and restoration efforts. In some places, populations are so degraded and patchy that attaining access to an effective number of genetically diverse, sexually mature colonies can be challenging. Thus, sexually mature restored populations can become spawning hubs that contribute to both natural population recovery and managed breeding efforts on land.
Questions remain, but science provides more than just hope
Despite our initial success, we still have many questions left to answer. For example, is bigger always better, or can we achieve the same outcome in less than five years and with smaller fused outplants? When it comes to disease susceptibility, research has shown that an increase in size can also mean an increased risk of disease. With our restoration sites now a part of the SCTLD endemic zone, which stretches from north of West Palm Beach down the southeast coast of Florida and through the entirety of the Florida Keys, this is something worth considering. We are also waiting to determine when the four other slow-growing coral species that we’ve applied this outplanting methodology to will reach sexual maturity and are curious to know how outcomes may differ by species. We’ve already seen fusion among the replicate fragments for some of the other species and are excited to see when they will spawn in the coming years.
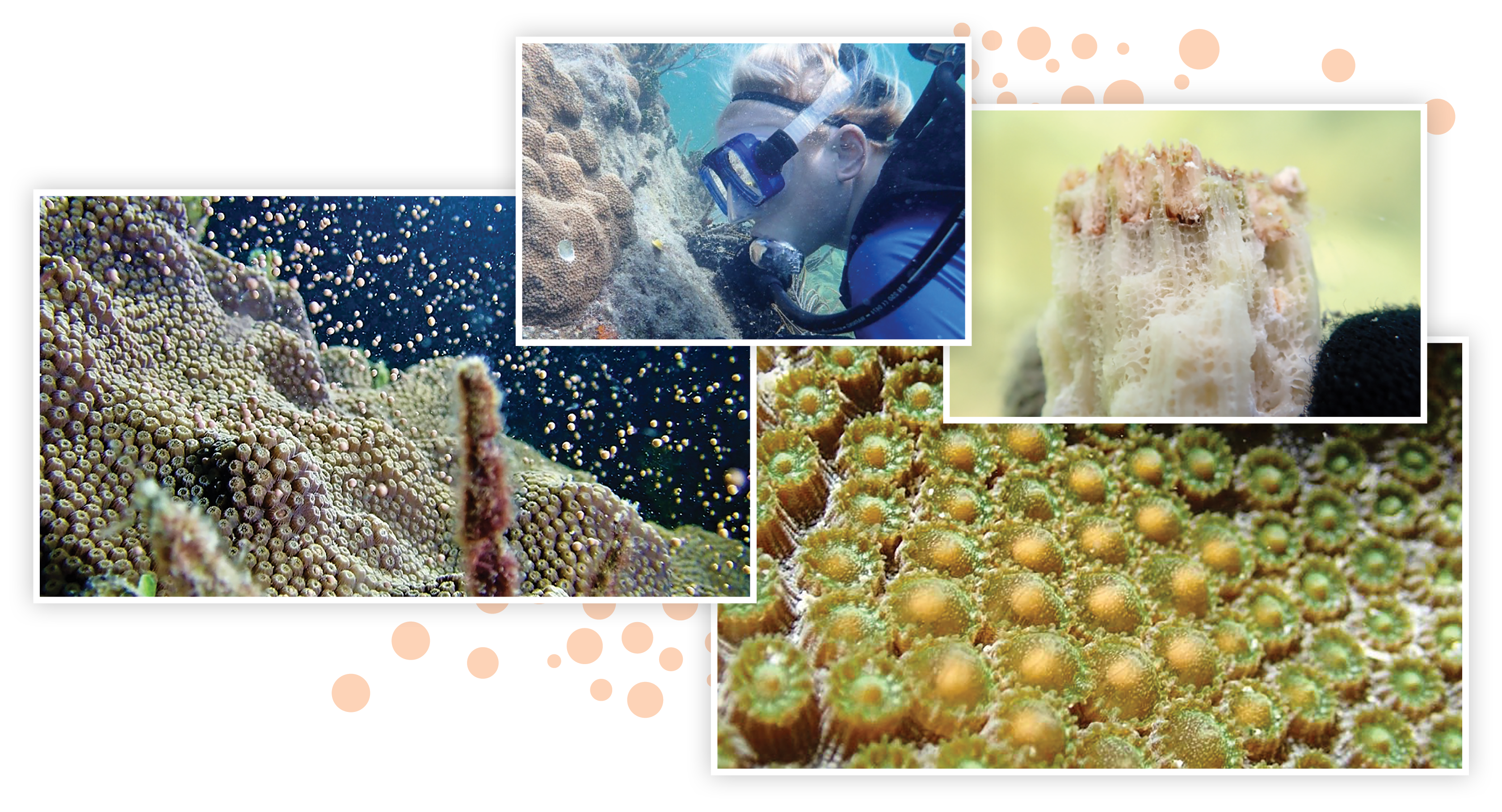
Finally, we will begin investigating the latter stages of the sexual cycle for these outplants, including confirming that new baby corals show up on the reef and contribute to the adult population. Ultimately, these processes are necessary for natural population recovery to take place. But that requires consideration of other mitigating factors such as water quality, suitable habitat availability, and ecosystem dynamics, including the presence of certain grazers and the prevalence of competitors.
For the best possible ecological outcomes, coral restoration should be combined with other measures such as habitat protection. Restoration strategies must also take into consideration the genetic consequences of their design and implementation. The methods with which practitioners select, rear, propagate, and manipulate corals for restoring degraded reefs will have consequences for the survivorship of outplants and resilience of restored populations. Understanding the genetic and demographic properties that influence the ability of populations to adapt to rapidly changing selective pressures will help practitioners design and implement optimal strategies. Finally, to mitigate the negative impacts of rapid climate change on coral reef ecosystems, carbon emissions need to be reduced.
Despite the challenges that lie ahead, there is reason for hope. For the first time in a long time, we have research that suggests the world’s corals can recover from the devastation they’ve endured—with some help. Already, hundreds of millions of dollars are being devoted to coral reef restoration around the world. Now, we’ve demonstrated a way to successfully invest those funds: a microfragmentation-fusion approach that provides the basis for quickly restoring coral populations to a sexually mature, potentially self-sustaining state, fundamentally changing the paradigm for coral restoration science.
Hanna R. Koch is a Mote Postdoctoral Research Fellow at Mote Marine Laboratory’s Elizabeth Moore International Center for Coral Reef Research & Restoration in Summerland Key, Florida. Mote Senior Scientist Erinn Muller is Manager of both the Coral Health & Disease Research Program and the Coral Restoration Program. Michael P. Crosby is a Senior Scientist and the president & CEO of Mote Marine Laboratory and Aquarium.
Interested in reading more?
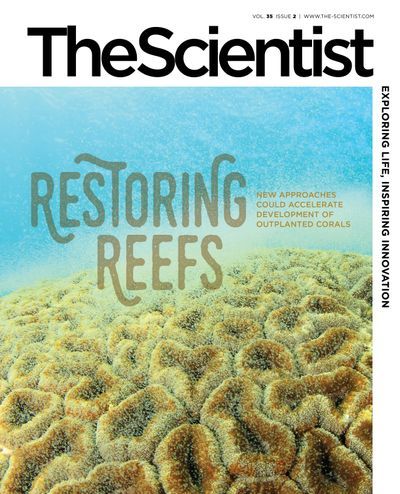