The Large Hadron Collider: Inside CERN's atom smasher
The Large Hadron Collider is the world's biggest particle accelerator.
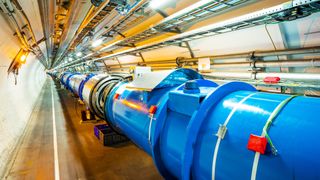
The Large Hadron Collider (LHC) is the biggest and most powerful particle accelerator in the world. It is located at the European particle physics laboratory CERN, in Switzerland.
The LHC restarted on April 22, 2022, after three years of maintenance work and upgrades. Run 3 is expected to commence on July, 5, a day after the 10-year anniversary of the Higgs boson discovery.
Scientists use the LHC to test theoretical predictions in particle physics, particularly those associated with the "Standard Model". While the Standard Model can explain almost all results in particle physics there are some questions left unanswered such as what is dark matter and dark energy? Why is there more matter than antimatter? The LHC is designed to help answer such questions.
The LHC can reproduce the conditions that existed within a billionth of a second of the Big Bang. The colossal accelerator allows scientists to collide high-energy subatomic particles in a controlled environment and observe the interactions. One of the most significant LHC breakthroughs came in 2012 with the discovery of the Higgs Boson.
Related: The Higgs boson could have kept our universe from collapsing
If you see a news headline about exotic new subatomic particles, the chances are the discovery was made at CERN, the European Organization for Nuclear Research, located near Geneva in Switzerland.
A recent example occurred in January 2022, when CERN scientists announced "evidence of X particles in the quark-gluon plasma produced in the Large Hadron Collider." Hiding behind that technospeak is the eye-popping fact that CERN succeeded in recreating a situation that hasn't occurred naturally since a few microseconds after the Big Bang.
When Run 3 commences we can expect a whole new spate of discoveries, so it's a good time to take a closer look at what makes the LHC — and the rest of CERN — so unique.
What is the Large Hadron Collider?
The LHC is a particle accelerator — a device that boosts subatomic particles to enormous energies in a controlled way so that scientists can study the resulting interactions, according to the CERN LHC fact sheet. The 'large' that the L stands for is an understatement; the LHC is by far the biggest accelerator in the world right now, occupying a circular tunnel nearly 17 miles (27 kilometers) in circumference. The middle letter, H, stands for 'hadron', the generic name for composite LHC particles such as protons that are made up of smaller particles called quarks. Finally, the C stands for 'collider' — the LHC accelerates two particle beams in opposite directions, and all the action takes place when the beams collide.
Like all physics experiments, the LHC aims to test theoretical predictions — in this case, the so-called Standard Model of particle physics — and see if there are any holes in them. As strange as it sounds, physicists are itching to find a few holes in the Standard Model because there are some things, such as dark matter and dark energy, that can't be explained until they do.
Large Hadron Collider discoveries and history
The LHC's biggest moment came in 2012 with the discovery of the Higgs boson. Although widely referred to as the "God particle", it's not really as awesome in itself as that name might suggest. Its huge significance came from the fact that it was the last prediction of the Standard Model that hadn't yet been proven. But the Higgs boson is far from being the LHC's only discovery.
According to the physics magazine CERN Courier, the LHC has also found around 60 previously unknown hadrons, which are complex particles made up of various combinations of quarks. Even so, all those new particles still lie within the bounds of the Standard Model, which the LHC has struggled to move beyond, much to the disappointment of the numerous scientists who have spent their careers working on alternative theories.
Related: 10 mind-boggling things you should know about quantum physics
The first tantalizing hints that a breakthrough might be just around the corner came in 2021 when analysis of LHC data revealed patterns of behavior that indicated small but definite departures from the Standard Model.
According to CERN, the LHC opened for business in 2009, but CERN's history goes back much further than that. The organization was established in 1954 following a recommendation by the European Council for Nuclear Research — or Conseil Européen pour la Recherche Nucléaire in French, from which it gets its name. Between its creation and the opening of the LHC, CERN was responsible for a series of groundbreaking discoveries, including weak neutral currents, light neutrinos and the W and Z bosons. As soon as the LHC is back up and running, we can expect discoveries to continue.
Run 3: What to expect
As the name suggests, Run 3 is the third science run of the LHC and will begin on July 5, 2022. It will build on LHC's discoveries made during its Run 1 (2009-2013) and Run 2 (2015 to 2018) and perform experiments through 2024.
On the precipice of new physics, scientists are keen to make use of the LHC's new upgrades to investigate the Higgs boson, explore dark matter and potentially expand our understanding of the standard model, the leading theory describing all known fundamental forces and elementary particles in the universe.
With the new upgrades, CERN has increased the power of the LHC's injectors, which feed beams of accelerated particles into the collider. At the time of the previous shutdown in 2018, the collider could accelerate beams up to an energy of 6.5 teraelectronvolts, and that value has been raised to 6.8 teraelectronvolts, according to a statement from CERN.
For reference, a single teraelectronvolt is equivalent to 1 trillion electron volts (an electron volt, a unit of energy, is equivalent to the work done on an electron accelerating through the potential of one volt.)
To increase the energy of the proton beams to such an extreme level, "the thousands of superconducting magnets, whose fields direct the beams around their trajectory, need to grow accustomed to much stronger currents after a long period of inactivity during LS2," the same CERN statement read. Getting the equipment up to speed in this upgrade is a process that CERN calls "magnet training" and which is made up of about 12,000 individual tests.
With LHC's magnets "trained" and the proton beams more powerful than ever, the LHC will be able to create collisions at higher energies than ever before, expanding the possibilities for what scientists using the upgraded equipment might find.
Once Run 3 concludes in 2024, CERN scientists will shut it down for another planned overhaul that will include more upgrades for the massive particle accelerator. Once complete, those upgrades will allow scientists to rename LHC the "High Luminosity Large Hadron Collider" once it reopens in 2028.
How does the Large Hadron Collider work?
As huge as it is, the LHC can't function without the help of other machines around it. Before particles, which are usually protons but for some experiments are much heavier lead ions, are injected into it, they're passed through a chain of smaller accelerators that progressively boost their speed, according to a CERN LHC report. Smaller is just a relative term; the last step in the injector chain, the Super Proton Synchrotron, is almost 4.3 miles in circumference (6.9 km). The result is two beams traveling in opposite directions around the LHC at virtually the speed of light, according to CERN.
The beams are kept on their circular trajectories by a strong magnetic field, which has the effect of bending the path of electrically charged particles. At four points around the LHC's vast ring, the opposing beams are brought together and made to collide, and that's where all the science happens.
Particles are smashed together with such enormous energies that the collisions create a cascade of new particles — most of them extremely short-lived. The important thing for scientists is to work out what all these particles are, and that's not an easy task.
The LHC has an array of sophisticated particle detectors for this purpose, each made up of layers of subdetectors designed to measure certain particle properties or to look for specific types of particles. For example, calorimeters measure a particle's energy, while the curving track of a particle in a magnetic field reveals information about its electric charge and momentum.
Two of the four collision points around the circumference of the LHC are occupied by large general-purpose detectors. These include the Compact Muon Solenoid (CMS), which can be thought of as a giant 3D camera, snapping images of particles up to 40 million times per second.
The paths of the particles inside the detector are controlled by a gigantic electromagnet called a solenoid. Despite weighing 12,500 metric tons, it's quite compact, as the detector's name suggests. That middle word, muon, refers to an elusive particle similar to the electron but much more massive, which requires its array of subdetectors wrapped around the solenoid.
The LHC's other general-purpose detector, ATLAS (A Toroidal LHC Apparatus), has an identical purpose to CMS but differs in the design of its detection, subsystems and magnets. It's also less compact than CMS, occupying a greater volume than any other particle detector ever built.
Large Hadron Collider experiments
Many of the LHC's most important experiments, including the discovery of the Higgs boson, utilize the general-purpose detectors ATLAS and CMS. But it also has several other more specialized detectors that can be used in specific types of experiments.
The LHC forward (LHCf) detector, located close to the ATLAS interaction point, uses particles thrown forward in collisions as a means of simulating cosmic rays under laboratory conditions. Further, along the beam trajectory is the Forward Search Experiment (FASER), designed to look for light, weakly interacting particles that are likely to elude the larger detectors.
A third experiment optimized for the forward direction is Total Elastic and diffractive cross-section Measurement (TOTEM), located near the CMS interaction point, which focuses on the physics of the high-energy protons themselves.
Away from ATLAS and CMS, the LHC has two other interaction points. One is occupied by A Large Ion Collider Experiment (ALICE), a specialized detector for heavy-ion physics. The final interaction point is home to two experiments on the very cutting edge of physics: LHCb, devoted to the physics of the exotic 'beauty quark', and MoEDAL — the Monopole and Exotics Detector at the LHC.
LHC and the Higgs boson
According to CERN, when physicists come up with new theories, they always try to make sure they can be tested experimentally. That happened in the early 1960s when Peter Higgs and others developed a theory to explain why certain force-carrier particles have non-zero mass.
The theory predicted the existence of a previously unsuspected particle, dubbed the Higgs boson. The next step was to find the Higgs boson and thus validate the theory. As simple as that sounds, it led to a decades-long hunt around the world. The end finally came in 2012, when data from the LHC — specifically, from a combination of ATLAS and CMS measurements — proved beyond doubt that the Higgs boson had been discovered.
CERN's many experiments
One of the key mysteries of the universe is the striking asymmetry between matter and antimatter — why it contains so much more of the former than the latter. According to the Big Bang theory, the universe must have started with equal amounts of both. Yet very early on, probably within the first second, virtually all the antimatter had disappeared, and only the normal matter we see today was left. This asymmetry has been given the technical name 'CP violation', and studying it is one of the main aims of the Large Hadron Collider's LHCb experiment.
All hadrons are made up of quarks, but LHCb is designed to detect particles that include a particularly rare type of quark known as 'beauty'. Studying CP violation in beauty-containing particles is one of the most promising ways to shed light on the emergence of matter-antimatter asymmetry in the early universe.
Hunting exotic particles
Sharing the same underground cavern as LHCb is a smaller instrument called MoEDAL, which stands for "Monopole and Exotics Detector at the LHC". While most CERN experiments are designed to study known particles, this one is aimed at discovering hitherto unknown ones that lie outside the present Standard Model. A monopole, for example, would be a magnetized particle consisting only of a north pole without a south one, or vice versa. Such particles have long been hypothesized, but never observed.
The purpose of MoEDAL is to look out for any monopoles that might be created in collisions inside the LHC. It could also potentially detect certain "stable massive particles" that are predicted by theories beyond the Standard Model. If it's successful in finding any of these particles, MoEDAL could help to resolve fundamental questions such as the existence of other dimensions or the nature of dark matter.
Climate science
Away from the LHC, there are other facilities at CERN that are doing equally important research. Linking particle physics to climate science may not be an obvious step, yet that's what one experiment is doing at CERN's Proton Synchrotron. This is a smaller and less sophisticated accelerator than the LHC, but it's still capable of doing useful work.
The climate experiment is called CLOUD, which gives a strong hint of what it's about, although the name stands for Cosmics Leaving Outdoor Droplets. Earth is under constant bombardment by cosmic rays, and it's been theorized that these play a role in cloud formation by seeding tiny water droplets. It isn't an easy process to study in the real atmosphere with real cosmic rays, so CERN is creating its own cosmic rays with the accelerator. These are then fired into an artificial atmosphere, where their effects can be studied much more closely.
Making antimatter
Antimatter often pops into existence inside CERN’s high-energy accelerators, as one-half of a particle-antiparticle pair. But in the usual course of events, the antiparticles don’t last long before they’re annihilated in collisions with ordinary particles.
If you want to create antimatter that stays around long enough for detailed study, you need more than just an accelerator. This is where CERN's unique “antimatter factory” comes in. It takes antiparticles created in the Proton Synchrotron and slows them down to manageable speeds in what is effectively the exact opposite of a particle accelerator: the Antiproton Decelerator. The resulting "anti-atoms" can then be studied by a range of instruments such as AEGIS (Antihydrogen Experiment: Gravity, Interferometry and Spectroscopy).
One question that AEGIS should be able to answer soon is the fascinating one of whether antimatter falls downwards in a gravitational field, like ordinary matter, or upwards in the opposite direction.
Is the Large Hadron Collider dangerous?
For various reasons over the years, people have speculated that experiments at CERN might pose a danger to the public. Fortunately, such worries are groundless. Take for example the N in CERN, which stands for "nuclear", according to UK Research and Innovation (UKRI). This has nothing to do with the reactions that take place inside nuclear weapons, which involve swapping protons and neutrons inside nuclei.
CERN's research is at an even lower level than this, in the constituents of the protons and neutrons themselves. It's sometimes referred to as "high energy" physics, but the energies are only "high" when viewed on a subatomic scale. Particles inside the LHC, for example, typically only have the energy of a mosquito, according to the LHC Safety Assessment Group's safety report.
People have also worried that the LHC might produce a "mini black hole," but even if this happened — which is unlikely — it would be unbelievably tiny, and so unstable that it would vanish within a fraction of a second according to the safety report. report.
Beyond the Large Hadron Collider
Over 12 years after it entered service, the LHC is still the world's biggest and most powerful particle accelerator. But it won't hold that record forever. Several countries have plans to go a step further, including China's Circular Electron Positron Collider and the International Linear Collider in Japan.
Europe's proposal is the Future Circular Collider (FCC), to be built near the LHC at CERN but dwarfing it in size. Though not yet financially approved — the estimated cost is £20 billion ($27 billion) — the design is well advanced according to Physics World.
The FCC would be 62 miles (99 km) in circumference and sit alongside the LHC, which it would use as a particle injector, ultimately achieving energies seven times greater than its predecessor.
Q&A with CERN scientist Clara Nellist
Dr. Nellist works on the Large Hadron Collider's ATLAS experiment at CERN.
We discuss what it's like to work with the world's largest particle accelerator.
How did you come to be involved with the ATLAS experiment?
I started on ATLAS for my PhD research. I was developing new pixel sensors to improve the measurement of particles as they pass through our detector. It's really important to make them resistant to radiation damage, which is a big concern when you put the sensors close to the particle collisions. Since then, I've had the opportunity to work on a number of different projects, such as understanding how the Higgs boson and the top quark interact with each other. Now I'm applying machine learning algorithms to our data to look for hints of dark matter. One of the biggest mysteries in physics right now is, what is 85% of the matter in our universe? We call it dark matter, but we don't actually know much about it!
What's it like working with such a unique and powerful machine?
It's really amazing to be able to work on this incredibly complicated machine with people from all over the world. No one person can run it all, so each team becomes an expert on their specific part. When we all work together, we can make discoveries about the smallest building blocks of our universe.
Are there any exciting new developments you're particularly looking forward to?
We're starting the Large Hadron Collider up again this year, so I'm really excited to see what we might find with it. Part of our work is to understand the particles we already know about in as much detail as possible to check that our theories match what we measure. But we're also looking for brand-new particles that we've never seen before. If we find something new, it could be a candidate for dark matter, or it could be something completely unexpected.
Additional resources
You can take a virtual tour of the Large Hadron Collider with the European Council for Nuclear Research (CERN), which gives you a 360-degree look inside the collider. You can also view the status of the Large Hadron Collider in real-time with CERN's Vistar tool. Learn about what particle accelerators have done for us in this interesting article from Physics World. There are many particle accelerators all around the world, for a comprehensive list of examples, check out this resource from the Physics Institute of the University of Bonn, Germany.
Bibliography
- Sirunyan, A. M., et al. "Evidence for X (3872) in Pb-Pb Collisions and Studies of its Prompt Production at s N N= 5.02 TeV." Physical Review Letters 128.3 (2022): 032001.
- Aaij, Roel, et al. "Test of lepton universality in beauty-quark decays." arXiv preprint arXiv:2103.11769 (2021).
- LHC Safety Assessment Group "Review of the Safety of LHC Collisions".
- LHC Safety Assessment Group "Review of the Safety of LHC Collisions Addendum on strangelets". June 2008.
- Giddings, Steven B., and Michelangelo L. Mangano. "Astrophysical implications of hypothetical stable TeV-scale black holes." Physical Review D 78.3 (2008): 035009.
- Aad, Georges, et al. "The ATLAS experiment at the CERN large hadron collider." Journal of instrumentation 3.S08003 (2008).
- Dimopoulos, Savas, and Greg Landsberg. "Black holes at the large hadron collider." Physical Review Letters 87.16 (2001): 161602.
Join our Space Forums to keep talking space on the latest missions, night sky and more! And if you have a news tip, correction or comment, let us know at: [email protected].
Get the Space.com Newsletter
Breaking space news, the latest updates on rocket launches, skywatching events and more!
Andrew May holds a Ph.D. in astrophysics from Manchester University, U.K. For 30 years, he worked in the academic, government and private sectors, before becoming a science writer where he has written for Fortean Times, How It Works, All About Space, BBC Science Focus, among others. He has also written a selection of books including Cosmic Impact and Astrobiology: The Search for Life Elsewhere in the Universe, published by Icon Books.