Stars: Facts about stellar formation, history and classification
How are stars named? And what happens when they die? These star facts explain the science of the night sky.
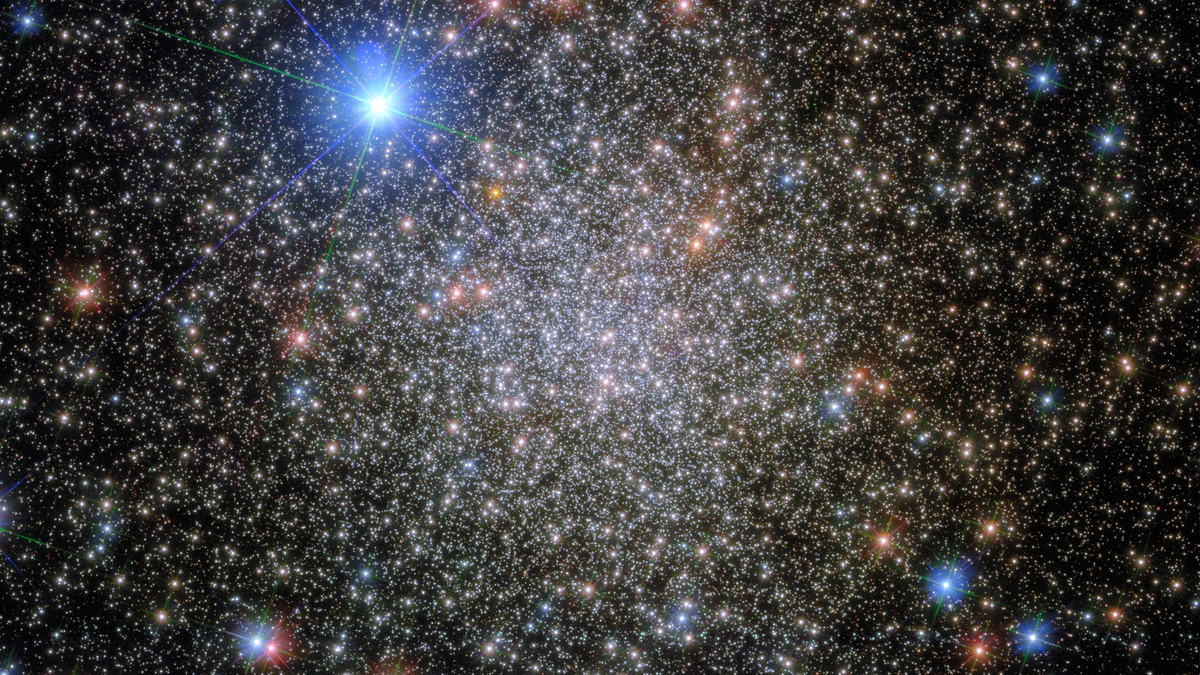
Stars are giant, luminous spheres of plasma. There are billions of them — including our own sun — in the Milky Way galaxy. And there are billions of galaxies in the universe. So far, we have learned that hundreds of stars also have planets orbiting them.
How are stars formed?
A star develops from a giant, slowly rotating cloud that is made up entirely or almost entirely of hydrogen and helium. Due to its own gravitational pull, the cloud begins to collapse inward, and as it shrinks, it spins more and more quickly, with the outer parts becoming a disk while the innermost parts become a roughly spherical clump.
According to NASA, this collapsing material grows hotter and denser, forming a ball-shaped protostar. When the heat in the protostar reaches about 1.8 million degrees Fahrenheit (1 million degrees Celsius), atomic nuclei that normally repel each other start fusing together, and the star ignites. Nuclear fusion converts a small amount of the mass of these atoms into extraordinary amounts of energy — for instance, 1 gram of mass converted entirely to energy would be equal to an explosion of roughly 22,000 tons of TNT.
Stellar evolution
The life cycles of stars follow patterns based mostly on their initial mass. These include intermediate-mass stars such as the sun, with half to eight times the mass of the sun, high-mass stars that are more than eight solar masses, and low-mass stars a tenth to half a solar mass in size. The greater a star's mass, the shorter its lifespan generally is, according to NASA. Objects smaller than a tenth of a solar mass do not have enough gravitational pull to ignite nuclear fusion — some might become failed stars known as brown dwarfs.
An intermediate-mass star begins with a cloud that takes about 100,000 years to collapse into a protostar with a surface temperature of about 6,750 degrees F (3,725 degrees C). After hydrogen fusion starts, the result is a T-Tauri star, a variable star that fluctuates in brightness. This star continues to collapse for roughly 10 million years until its expansion due to energy generated by nuclear fusion is balanced by its contraction from gravity, after which point it becomes a main-sequence star that gets all its energy from hydrogen fusion in its core.
The greater the mass of such a star, the more quickly it will use its hydrogen fuel and the shorter it stays on the main sequence. After all the hydrogen in the core is fused into helium, the star changes rapidly — without nuclear radiation to resist it, gravity immediately crushes matter down into the star's core, quickly heating the star. This causes the star's outer layers to expand enormously and to cool and glow red as they do so, rendering the star a red giant.
Helium starts fusing together in the core, and once the helium is gone, the core contracts and becomes hotter, once more expanding the star but making it bluer and brighter than before, blowing away its outermost layers. After the expanding shells of gas fade, the remaining core is left, a white dwarf that consists mostly of carbon and oxygen with an initial temperature of roughly 180,000 degrees F (100,000 degrees C). Since white dwarves have no fuel left for fusion, they grow cooler and cooler over billions of years to become black dwarves too faint to detect. Our sun should leave the main sequence in about 5 billion years, according to Live Science.
A high-mass star forms and dies quickly. These stars form from protostars in just 10,000 to 100,000 years. While on the main sequence, they are hot and blue, some 1,000 to 1 million times as luminous as the sun and are roughly 10 times wider. When they leave the main sequence, they become a bright red supergiant and eventually become hot enough to fuse carbon into heavier elements. After some 10,000 years of such fusion, the result is an iron core roughly 3,800 miles (6,000 km) wide, and since any more fusion would consume energy instead of liberating it, the star is doomed, as its nuclear radiation can no longer resist the force of gravity.
When a star reaches a mass of more than 1.4 solar masses, electron pressure cannot support the core against further collapse, according to NASA. The result is a supernova. Gravity causes the core to collapse, making the core temperature rise to nearly 18 billion degrees F (10 billion degrees C), breaking the iron down into neutrons and neutrinos. In about one second, the core shrinks to about six miles (10 km) wide and rebounds just like a rubber ball that has been squeezed, sending a shock wave through the star that causes fusion to occur in the outlying layers. The star then explodes in a so-called Type II supernova. If the remaining stellar core was less than roughly three solar masses large, it becomes a neutron star made up nearly entirely of neutrons, and rotating neutron stars that beam out detectable radio pulses are known as pulsars. If the stellar core was larger than about three solar masses, no known force can support it against its own gravitational pull, and it collapses to form a black hole.
A low-mass star uses hydrogen fuel so sluggishly that they can shine as main-sequence stars for 100 billion to 1 trillion years — since the universe is only about 13.7 billion years old, according to NASA, this means no low-mass star has ever died. Still, astronomers calculate these stars, known as red dwarfs, will never fuse anything but hydrogen, which means they will never become red giants. Instead, they should eventually just cool to become white dwarfs and then black dwarves.
History of star observations
Since the dawn of recorded civilization, stars played a key role in religion and proved vital to navigation, according to the International Astronomical Union. Astronomy, the study of the heavens, may be the most ancient of the sciences. The invention of the telescope and the discovery of the laws of motion and gravity in the 17th century prompted the realization that stars were just like the sun, all obeying the same laws of physics. In the 19th century, photography and spectroscopy — the study of the wavelengths of light that objects emit — made it possible to investigate the compositions and motions of stars from afar, leading to the development of astrophysics.
In 1937, the first radio telescope was built, enabling astronomers to detect otherwise invisible radiation from stars. The first gamma-ray telescope launched in 1961, pioneering the study of star explosions (supernovae). Also in the 1960s, astronomers commenced infrared observations using balloon-borne telescopes, gathering information about stars and other objects based on their heat emissions; the first infrared telescope (the Infrared Astronomical Satellite) was launched in 1983.
Microwave emissions were first studied from space in 1992, with NASA's Cosmic Microwave Background Explorer (COBE) satellite. (Microwave emissions are generally used to probe the young universe's origins, but they are occasionally used to study stars.) In 1990, the first space-based optical telescope, the Hubble Space Telescope, was launched, providing the deepest, most detailed visible-light view of the universe.
There have been, of course, more advanced observatories (in all wavelengths) over the years, and even more powerful ones are planned. A couple of examples are the Extremely Large Telescope (ELT), which is planned to start observations in 2024 in infrared and optical wavelengths. Also, NASA's James Webb Space Telescope – billed as a successor to Hubble – will launch in 2018 to probe stars in infrared wavelengths.
How are stars named?
Ancient cultures saw patterns in the heavens that resembled people, animals or common objects — constellations that came to represent figures from myth, such as Orion the Hunter, a hero in Greek mythology.
Astronomers now often use constellations in the naming of stars. The International Astronomical Union, the world authority for assigning names to celestial objects, officially recognizes 88 constellations. Usually, the brightest star in a constellation has "alpha," the first letter of the Greek alphabet, as part of its scientific name. The second brightest star in a constellation is typically designated "beta," the third brightest "gamma," and so on until all the Greek letters are used, after which numerical designations follow.
A number of stars have possessed names since antiquity — Betelgeuse, for instance, means "the hand (or the armpit) of the giant" in Arabic. It is the brightest star in Orion, and its scientific name is Alpha Orionis. Also, different astronomers over the years have compiled star catalogs that use unique numbering systems. The Henry Draper Catalog, named after a pioneer in astrophotography, provides spectral classification and rough positions for 272,150 stars and has been widely used by the astronomical community for over half a century. The catalog designates Betelgeuse as HD 39801.
Since there are so many stars in the universe, the IAU uses a different system for newfound stars. Most consist of an abbreviation that stands for either the type of star or a catalog that lists information about the star, followed by a group of symbols. For instance, PSR J1302-6350 is a pulsar, thus the PSR. The J reveals that a coordinate system known as J2000 is being used, while the 1302 and 6350 are coordinates similar to the latitude and longitude codes used on Earth.
In recent years, the IAU formalized several names for stars amid calls from the astronomical community to include the public in their naming process. The IAU formalized 14 star names in the 2015 "Name ExoWorlds" contest, taking suggestions from science and astronomy clubs around the world.
Then in 2016, the IAU approved 227 star names, mostly taking cues from antiquity in making its decision. The goal was to reduce variations in star names and also spelling ("Formalhaut", for example, had 30 recorded variations.) However, the long-standing name "Alpha Centauri" – referring to a famous star system with planets just four light-years from Earth – was replaced with Rigel Kentaurus.
Binary stars and other multiples
Although our solar system only has one star, most stars like our sun are not solitary but are binaries, where two stars or multiple stars orbit each other. In fact, just one-third of stars like our sun are single, while two-thirds are multiples — for instance, the closest neighbor to our solar system, Proxima Centauri, is part of multiple systems that also includes Alpha Centauri A and Alpha Centauri B. Still, class G stars like our sun only make up some 7% of all the stars we see — when it comes to systems in general, about 30 percent in our galaxy are multiple, while the rest are single, according to Charles J. Lada of the Harvard-Smithsonian Center for Astrophysics.
Binary stars develop when two protostars form near each other. One member of this pair can influence its companion if they are close enough together, stripping away matter in a process called mass transfer. If one of the members is a giant star that leaves behind a neutron star or a black hole, an X-ray binary can form, where matter pulled from the stellar remnant's companion can get extremely hot — more than 1 million F (555,500 C) and emit X-rays.
If a binary includes a white dwarf, gas pulled from a companion onto the white dwarf's surface can fuse violently in a flash called a nova. At times, enough gas builds up for the dwarf to collapse, leading its carbon to fuse nearly instantly and the dwarf to explode in a Type I supernova, which can outshine a galaxy for a few months.
Key characteristics of stars
Brightness
Astronomers describe star brightness in terms of magnitude and luminosity.
The magnitude of a star is based on a scale more than 2,000 years old, devised by Greek astronomer Hipparchus around 125 BC, according to NASA's Ask and Astrophysicist. He numbered groups of stars based on their brightness as seen from Earth — the brightest stars were called first magnitude stars, the next brightest were the second magnitude, and so on up to sixth magnitude, the faintest visible ones.
Nowadays astronomers refer to a star's brightness as viewed from Earth as its apparent magnitude, but since the distance between Earth and the star can affect the light one sees from it, they now also describe the actual brightness of a star using the term absolute magnitude, which is defined by what its apparent magnitude would be if it were 10 parsecs or 32.6 light-years from Earth. The magnitude scale now runs to more than six and less than one, even descending into negative numbers — the brightest star in the night sky is Sirius, with an apparent magnitude of -1.46.
Luminosity is the power of a star — the rate at which it emits energy. Although power is generally measured in watts — for instance, the sun's luminosity is 400 trillion trillion watts— the luminosity of a star is usually measured in terms of the luminosity of the sun. For example, Alpha Centauri A is about 1.3 times as luminous as the sun. To figure out luminosity from absolute magnitude, one must calculate that a difference of five on the absolute magnitude scale is equivalent to a factor of 100 on the luminosity scale — for instance, a star with an absolute magnitude of 1 is 100 times as luminous as a star with an absolute magnitude of 6.
The brightness of a star depends on its surface temperature and size.
Color
Stars come in a range of colors, from reddish to yellowish to blue. The color of a star depends on surface temperature.
A star might appear to have a single color, but actually emits a broad spectrum of colors, potentially including everything from radio waves and infrared rays to ultraviolet beams and gamma rays. Different elements or compounds absorb and emit different colors or wavelengths of light, and by studying a star's spectrum, one can divine what its composition might be.
Surface temperature
Astronomers measure star temperatures in a unit known as the kelvin, with a temperature of zero K ("absolute zero") equaling minus 273.15 degrees C, or minus 459.67 degrees F. A dark red star has a surface temperature of about 2,500 K (2,225 C and 4,040 F); a bright red star, about 3,500 K (3,225 C and 5,840 F); the sun and other yellow stars, about 5,500 K (5,225 C and 9,440 F); a blue star, about 10,000 K (9,725 C and 17,540 F) to 50,000 K (49,725 C and 89,540 F).
The surface temperature of a star depends in part on its mass and affects its brightness and color. Specifically, the luminosity of a star is proportional to temperature to the fourth power. For instance, if two stars are the same size but one is twice as hot as the other in kelvin, the former would be 16 times as luminous as the latter.
Related: How hot is the sun?
Size
Astronomers generally measure the size of stars in terms of the radius of our sun. For instance, Alpha Centauri A has a radius of 1.05 solar radii (the plural of radius). Stars range in size from neutron stars, which can be only 12 miles (20 kilometers) wide, to supergiants roughly 1,000 times the diameter of the sun.
The size of a star affects its brightness. Specifically, luminosity is proportional to radius squared. For instance, if two stars had the same temperature, if one star was twice as wide as the other one, the former would be four times as bright as the latter.
Mass
Astronomers represent the mass of a star in terms of the solar mass, the mass of our sun. For instance, Alpha Centauri A is 1.08 solar masses.
Stars with similar masses might not be similar in size because they have different densities. For instance, Sirius B is roughly the same mass as the sun but is 90,000 times as dense, and so is only a fiftieth its diameter.
The mass of a star affects surface temperature.
Magnetic field
Stars are spinning balls of roiling, electrically charged gas, and thus typically generate magnetic fields. When it comes to the sun, researchers have discovered its magnetic field can become highly concentrated in small areas, creating features ranging from sunspots to spectacular eruptions known as flares and coronal mass ejections. A survey at the Harvard-Smithsonian Center for Astrophysics found that the average stellar magnetic field increases with the star's rate of rotation and decreases as the star ages.
Metallicity
The metallicity of a star measures the amount of "metals" it has — that is, any element heavier than helium.
Three generations of stars may exist based on metallicity. Astronomers have not yet discovered any of what should be the oldest generation, Population III stars born in a universe without "metals." When these stars died, they released heavy elements into the cosmos, which Population II stars incorporated relatively small amounts of. When a number of these died, they released more heavy elements, and the youngest Population I stars like our sun contain the largest amounts of heavy elements.
Star classification
Stars are typically classified by their spectrum in what is known as the Morgan-Keenan or MK system, according to the European Southern Observatory. There are eight spectral classes, each analogous to a range of surface temperatures — from the hottest to the coldest, these are O, B, A, F, G, K, M and L. Each spectral class also consists of 10 spectral types, ranging from the numeral 0 for the hottest to the numeral 9 for the coldest.
Stars are also classified by their luminosity under the Morgan-Keenan system. The largest and brightest classes of stars have the lowest numbers, given in Roman numerals — Ia is a bright supergiant; Ib, a supergiant; II, a bright giant; III, a giant; IV, a subgiant; and V, a main sequence or dwarf.
A complete MK designation includes both spectral type and luminosity class — for instance, the sun is a G2V.
Stellar structure
The structure of a star can often be thought of as a series of thin nested shells, somewhat like an onion.
A star during most of its life is a main-sequence star, which consists of a core, radiative and convective zones, a photosphere, a chromosphere and a corona. The core is where all the nuclear fusion takes place to power a star.
In the radiative zone, energy from these reactions is transported outward by radiation, like heat from a light bulb, while in the convective zone, energy is transported by the roiling hot gases, like hot air from a hairdryer. Massive stars that are more than several times the mass of the sun are convective in their cores and radiative in their outer layers, while stars comparable to the sun or less in mass are radiative in their cores and convective in their outer layers. Intermediate-mass stars of spectral type A may be radiative throughout.
After those zones come the part of the star that radiates visible light, the photosphere, which is often referred to as the surface of the star. After that is the chromosphere, a layer that looks reddish because of all the hydrogen found there. Finally, the outermost part of a star's atmosphere is the corona, which if super-hot might be linked with convection in the outer layers.
Additional resources
To explore the stars of our universe for yourself, you can use NASA's Skymap tool. Additionally, to see images of stars taken by the Hubble Space Telescope, browse the European Space Agency's (ESA) image archive.
Bibliography
Salaris, M., & Cassisi, S. (2005). "Evolution of stars and stellar populations". John Wiley & Sons. https://books.google.co.uk
"Spectral Classification". Annual Review of Astronomy and Astrophysics (1973). https://www.annualreviews.org/doi/abs/10.1146/annurev.aa.11.090173.000333?journalCode=astro
"The Gas–Star Formation Cycle in Nearby Star-forming Galaxies. I. Assessment of Multi-scale Variations". The Astrophysical Journal (2019). https://iopscience.iop.org/article/10.3847/1538-4357/ab50c2/meta
Join our Space Forums to keep talking space on the latest missions, night sky and more! And if you have a news tip, correction or comment, let us know at: [email protected].
Get the Space.com Newsletter
Breaking space news, the latest updates on rocket launches, skywatching events and more!
Charles Q. Choi is a contributing writer for Space.com and Live Science. He covers all things human origins and astronomy as well as physics, animals and general science topics. Charles has a Master of Arts degree from the University of Missouri-Columbia, School of Journalism and a Bachelor of Arts degree from the University of South Florida. Charles has visited every continent on Earth, drinking rancid yak butter tea in Lhasa, snorkeling with sea lions in the Galapagos and even climbing an iceberg in Antarctica. Visit him at http://www.sciwriter.us