How Will We Know We’re Not Alone?
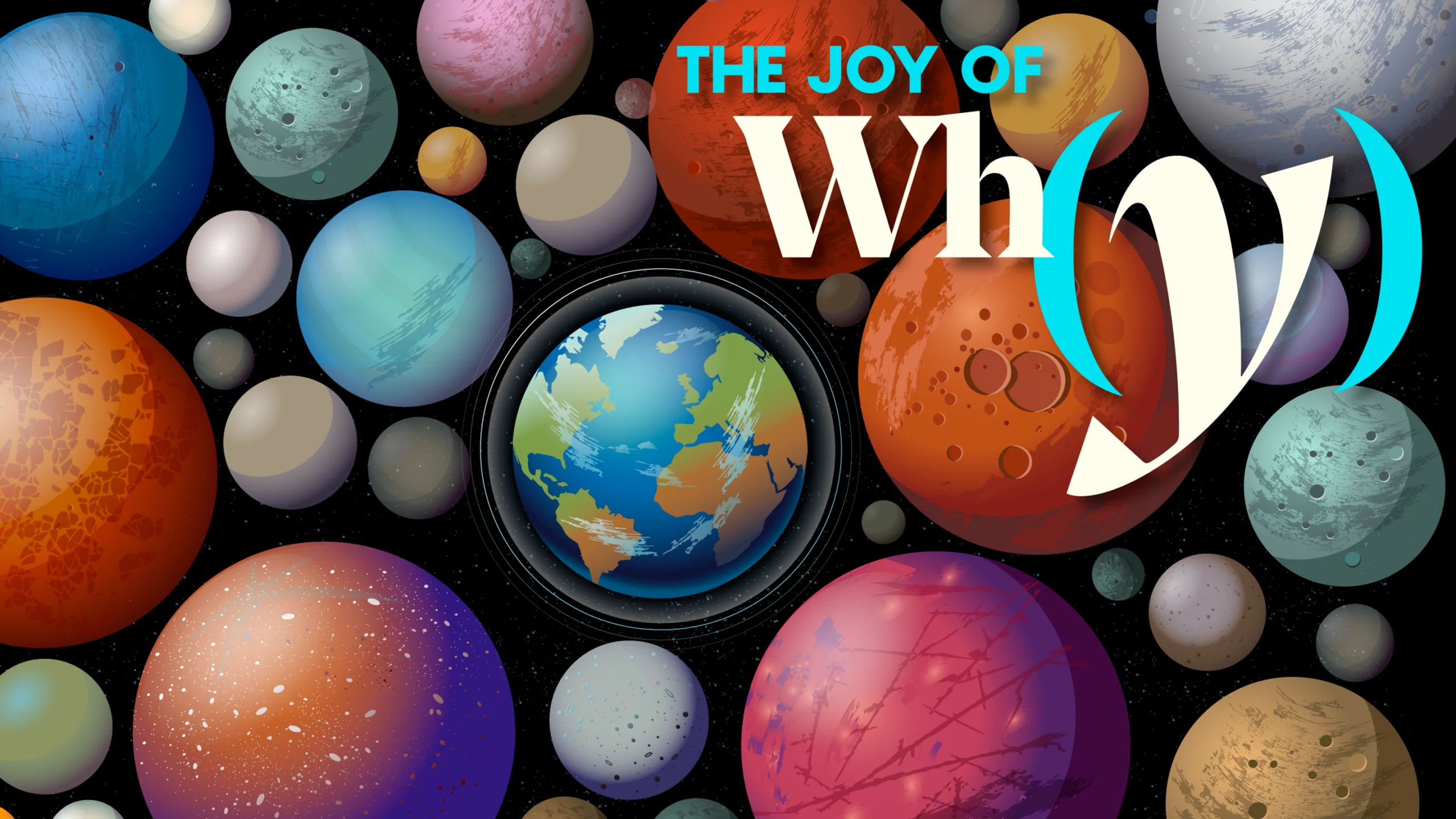
Peter Greenwood for Quanta Magazine
Introduction
We have identified thousands of planets just in our neighborhood in the Milky Way, mostly from the way they impact their host stars. Basic calculations suggest that there are countless more across the galaxy, and that billions of them could potentially support life. But what kind of life they host, and how we would be able to detect the presence of those biological processes from Earth, remain big questions in the world of exoplanets and astrobiology. What technologies might lie ahead to help us answer the question of whether we are alone in the universe? Lisa Kaltenegger, an astrophysicist and astrobiologist at Cornell University, talks to Janna Levin about that search, the atmospheric fingerprints of life, and why an advanced alien civilization might decide not to talk to us.
Listen on Apple Podcasts, Spotify, TuneIn or your favorite podcasting app, or you can stream it from Quanta.
Transcript
[Theme plays]
JANNA LEVIN: For millennia, ancient astronomers observed strange points of light that traced unusual patterns in the night. While most stars shifted collectively, a handful arced dramatically across the sky. The Greeks named these outliers “wandering stars,” or asteres planetai. Eventually, we’d realize these objects weren’t stars at all, but the term “planet” would stick.
As science progressed, we came to recognize that these planets orbit the sun, that our Earth is a planet, and only three decades ago, that planets, or exoplanets, exist outside our solar system.
Now astronomers estimate that there are trillions of exoplanets in our Milky Way galaxy alone, and with remarkable instruments like the James Webb Space Telescope, we’re able to detect other worlds more readily than ever before.
I’m Janna Levin, and this is “The Joy of Why,” a podcast from Quanta Magazine, where I take turns at the mic with my cohost, Steve Strogatz, exploring the biggest questions in math and science today.
[Theme ends]
In this episode, astrophysicist and astrobiologist Lisa Kaltenegger brings us along on the hunt for exoplanets and the quest to find alien life. Lisa is a professor of astronomy and the founding director of the Carl Sagan Institute at Cornell University. She’s a world-leading expert in modeling habitable worlds and is also the author of a new book, Alien Earths: The New Science of Planet Hunting in the Cosmos.
Lisa, thanks for joining us on “The Joy of Why.” So good to have you.
LISA KALTENEGGER: Thanks for having me.
LEVIN: I’ve really been looking forward to talking. This is a topic that has been of personal interest to me for a long time. The first convincing evidence of an extrasolar planet or an exoplanet was only confirmed fairly recently, 1995. That’s still recent in scientific terms. Now, why would we have thought before 1995 that it’s reasonable to presume that other planets exist in other solar systems? Why would that have been a premise before the observation?
KALTENEGGER: It was quite interesting because throughout history, there were always times when people said, “Oh, there must be other worlds.” And then it swapped into like, “There cannot be other worlds, there can only be us, because we’re unique and special.” And then the optimism took back over. There could be life on Venus; there could be life on Mars. There could be life in the cosmos around other stars.
I think as soon as we realized, as you so beautifully described, that these lights that are moving on our sky in this weird way are actually other planets around our sun, that idea started to take root. But trying to find a planet, especially a small one like our Earth around a bright, hot, huge star is incredibly challenging. It needed a while until the telescopes got big enough to spot them.
LEVIN: Now, what was the technique that was used back in the ’90s for detecting these?
KALTENEGGER: So that’s what we call a wobble technique, or radial velocity. And so you can see a star moves when you look at its light. So if the light is redder than it should be, then the object moves away from you. If it’s bluer than it should be, the object moves towards you.
And so when you see a star and the light of the star becomes too red and then it’s normal again, then it becomes too blue, then it’s normal again, then you know it’s wobbling. And if that wobble is very small, you know whatever is pulling on the star to make it move is a very small object. And small objects don’t shine themselves, they have no nuclear fusion in the core, and so that’s when we call them planets.
And so, the star and the planet do something in a way similar. The gravitational tug of the planet on the star makes the star move around And so in the wobble or in the movement of the star, we actually can spot that it’s not alone, that it has a companion. And that’s how they found the first exoplanet out there.
LEVIN: So, this wobble method yields a handful of planets, but the methods have changed a lot since then, haven’t they?
KALTENEGGER: They have, and that is exciting because that means we could find so many more planets. What we figured out a couple of years after is that if the planet goes close to its star, for some of them, we should actually see the star dim ever so slightly, because when the planet goes between our point of view to the star, you actually see it block out part of this hot stellar surface. And that happens again and again.
LEVIN: So it’s like a mini eclipse.
KALTENEGGER: It is basically exactly a mini eclipse. You have to be very careful looking at the star to not miss it, but once NASA put the Kepler satellite up in space, that looked at 150,000 stars for the slight dimming of light, and it found thousands of new worlds on our horizon.
And so now seven out of 10 new worlds we know out there have been found through this dimming of light. And yet we know they’re just the tip of the iceberg, because not every planet goes in front of their star from our point of view. So we know that what we find is just a fraction of what’s out there.
LEVIN: Now, Kepler managed to discover thousands of planets. So that was a huge leap. It was a difference in the technique, but also quite a massive jump in terms of the quantity of planets discovered. So how did that change our estimates of how many star systems we think will harbor planets?
KALTENEGGER: So the Kepler space mission was done so that we could figure out how many stars have a planet in the first place. And what it found is that about every star has a planet, like a huge Jupiter or it could be a small rocky world like our own. And it also figured out that every fifth star has a planet that is not too hot, not too close to the star, and not too cold, not too far away. So in what we call the habitable zone. And in our Milky Way, we have 200 billion stars in our own galaxy. So one out of five gives you billions of possibilities of potential other Earths out there. And that’s where it became so incredibly exciting because before, we didn’t know how many planets were out there, and we didn’t know how many small rocky worlds could be out there. And now we have the James Webb Space Telescope that can for the first time collect light from these small worlds to figure out if there are signs of a biosphere.
LEVIN: So let’s talk about that, there’s a lot to unpack there. The mirror on the James Webb is six times larger than the one on Hubble? Of course, it was this magnificent instrument where it had to unfold in space because the mirror was so unbelievably large. And what is the significance of having such a large mirror on Webb? Why is this instrument a huge leap even beyond the tremendous success of Kepler?
KALTENEGGER: In astronomy, when you get more light, you see more. And so the bigger our telescope, the more light we can collect from a very small and dim source — like a planet. And so if you have a big enough telescope, you can collect enough light to not just see a dot of light, but to split it up in its colors like a rainbow, and then you can have a look at that light fingerprint of another world and figure out what’s the chemical makeup of its atmosphere.
LEVIN: For the first time, there’s the prospect of actually looking at the planet itself, as opposed to indirectly inferring the existence of the planet from the effect it’s having on the star. Now you’re able to, because of this big collecting power, actually look at the planet itself. That’s pretty thrilling.
KALTENEGGER: It’s incredible. We were training for this with Hubble and with ground-based telescopes that could do this analysis for the big gaseous planets already, but we just didn’t get enough light for the small rocky worlds to do the same. And the way that I think about it is, if you were to shrink a planet like the Earth to the size of an apple, then the atmosphere — the thing we want to analyze — is thinner than the peel of that apple. So it is such a small signal that you’re trying to get. And this is why now is such an amazing time to live because we live in a golden era of exploration.
LEVIN: So this idea of a habitable zone and looking for other Earths, I wondered if that was falling out of favor. That our bias towards the Earth as the only kind of planet that’s going to support life because it supports our life — maybe that was fading, that people were starting to consider that the range of life could be beyond our imagination, and that we should take seriously that maybe life will occur on moons or maybe life will occur on a Jupiter-like atmosphere without a rocky surface. What do you think about that kind of twist?
KALTENEGGER: Oh, absolutely. Not even a question. Because in our solar system, we actually go to explore these icy moons like Europa and Enceladus where you have an ice crust and an ocean below it. And we hope that we’re going to be able to find life there. We have Titan, a very cold world with methane, ethane lakes, where we hope that liquid could take over the function of water because it’s just too cold on Titan. Water would freeze out so you’d need another liquid for life.
We think right now from the biology side of things, you want a surface and a liquid to get life started. Just the surface would help you to actually make a kind of protocell, something that can concentrate the chemistry. As soon as you can make a vesicle or some kind of container, then you can selectively let the chemistry in and out. So we think that happens when you have a surface where these different molecules can actually stick to and make more and more complex structures, like, for example, a cell.
LEVIN: That’s pretty fascinating.
KALTENEGGER: One of the problems that we have, of course, is we don’t know what life in this kind of different, bizarre chemistry could be like, and what signs it would leave that are unique in an atmosphere. The search for life is basically a search for signatures of a biosphere. And we have our own planet, the Earth, as our one example. And so the Earth has changed dramatically through its lifetime. So in the beginning there was no oxygen, the Earth was young, then oxygen starts to build up, and then at one point we come around. So the Earth, if you go back in time, is actually a lot of different kinds of planets. So this is our template to search.
And we keep our eyes open for weird signals, absolutely. But those weird signals, we won’t be able to tell if they’re geological, because geology can produce a lot of interesting gases and interesting colors. If we want to say we found signs, we also want to be able to say that there’s no other explanation than life. And so we’re becoming quite conservative. That means water and carbon, like on our own planet. But if you look around in the universe, you actually see carbon, hydrogen, and oxygen pretty much everywhere. And so, if you’re at the right distance from your star where water could be liquid, chances that the fluid could be water seem to be quite high.
LEVIN: So if I imagine that our solar system is roughly one light-year across, meaning the distance that light would travel in one year. And the Milky Way galaxy is about how large in terms of light years?
KALTENEGGER: So if you go from one side to the other, you have about a hundred thousand light years. So our search for these other worlds happens within about a thousand light years right now. The nearest star is a triple system, it’s about four light-years away. So we see that closest star to our sun like it was four years ago, roughly. So everything you see in the night sky has already happened, but we’re right now getting the information of what’s going on. If we go even further than our galactic borders, the further back in time we can look. And this is how we can see a young universe.
LEVIN: So even though we know that there are stars in the furthest reaches of the galaxies from where we’re located. We can’t tell if they have planets yet. It’s still too hard, so it’s really in the region around us. Still, a thousand light years is pretty close by in the scheme of things.
KALTENEGGER: Absolutely, it’s basically our cosmic neighborhood, but light expands in a sphere, so that means the further away from something that’s bright, the less light you get, And so that means the closer something is, even with the biggest telescope, the more light I can catch and the more I can tell about a planet — or about a star, and the smaller of a wobble I can find.
But there are some techniques who actually go further out, like the microlensing technique, where light gets bent because the masses in space-time bend the path of light. So there we can go further out and find planets, but those planets we cannot analyze because we cannot catch enough light of the atmosphere to figure out what they’re like. So I concentrate on the planets closer to us to be able to look at the light and figure out what’s in there.
LEVIN: Now, these systems that have been discovered, they can be quite, well, bizarre, only because we like ours, we’re used to ours. So it’s not as though, in these various exo-solar systems, that they come in a kind of template. They seem to be [of] great variety and even instabilities. Can you describe a little bit a fascinating system that you’ve seen that give us a sense of the sheer range of possibilities?
KALTENEGGER: So these systems are so incredibly diverse and were not what we’re expecting. Because in our system we have the four small rocky worlds close to the star where it was so hot that only the rocks were left over, and then further out where it was cool enough, you have the big gas planets. And that’s what we were expecting to find because that’s what we saw and that’s what we understood. And the first system ever, the 51 Pegasus B, is actually a Jupiter in a 4.5-day orbit. So we learned, even with the first system ever, that these massive, big gas balls can actually move. They can migrate and come closer to their star.
And ever since we found this first planet we have found a diversity that I don’t think anybody expected at all. We have hundreds and hundreds of systems of planets, because most planets don’t come alone. We find rocky worlds that are going around their stars in less than 18 hours. And we know it has to be so hot on those worlds that they’re covered in molten lava.
And then there are other worlds that are kind of between our biggest rock, the Earth, and the smallest gas planet, Neptune. We call them mini-Neptunes or super-Earths. So the most abundant planets that we found so far are some we don’t have in our solar system. And of course, a lot of those also go around double stars. So double sunsets and double sunrises are the norm on a lot of these worlds.
LEVIN: Wow, fascinating. Those are also presumably much more unstable than when you have just one star.
KALTENEGGER: The interesting thing there is that if we can spot them now, they have to be in a stable realm. We do get just a snapshot, but what’s interesting is that these planets, even if they go around two stars or around one of the two stars, there’s a stability region where they can basically sit and be happy ever after.
LEVIN: Now in anticipation of talking to you, I asked myself, do I know how many moons there are in our own solar system? And there are almost 300 known moons in our own solar system. I knew it was a large number, but that’s very large. Do we expect this to be a universal trend? A star system might have a handful of exoplanets and each one of those planets might have a handful of moons, or more than a handful?
KALTENEGGER: Absolutely. We have only our solar system to go on, but all of the giant planets have a huge amount of moons. And the ones that don’t have as many moons yet [are] the ones we haven’t sent a mission to, so we haven’t found all the moons yet because moons can be very small.
These gas giants, some of them sit smack in the middle of this warm temperate zone. And if they had a rocky moon, then that rocky moon could actually have the conditions to get life started. There could be billions and billions more of potential habitats out there just around these giant planets. But for now we don’t know, because they’re even harder to find, right? Because finding the signal of a planet is hard, but finding the signal of a moon around the planet signal that’s so hard to find is so much worse. So we don’t know yet.
[Break]
LEVIN: We’re speaking with astronomer Lisa Kaltenegger about how we can search for exoplanets around other stars and the proliferation of exoplanets and even exomoons.
And I think we’re dancing around what the real topic of excitement is. And that is the question of: Are we alone? Ultimately, a lot of the motivation about searching for exoplanets and exomoons is to search for life. And so how are you imagining going about the search for life besides just identifying planets in the right location made of the right rocky material? It seems that the subject’s progressed a lot.
KALTENEGGER: The subject has progressed a lot. When you look at Mars, or when you look at Venus, each planet in our own solar system has its unique light fingerprint. That means the light that the planet reflects, or that gets filtered through its atmosphere, tells you what the chemical makeup is in that planet. And the advantage we have is when the planet actually goes from our point of view in front of its star, part of the starlight gets filtered through the air of that planet. And when light hits a molecule, it can make that molecule swing and rotate. And because each molecule has a different structure, it needs a different energy to actually swing and rotate.
So, how can I figure out what’s in that air? I split up the light, I make a spectrum, and I see if there’s any energy missing. If there is, let’s say, water gas, and I shine the light of the star through, what light does not make it because it actually interacted with the molecules and made them swing and rotate? And so I’m providing myself with a comparison chart.
If the temperature is too hot or too cold, so my experimental setup would freeze or boil, or if the gases are poisonous, I do that with quantum mechanics, and I can actually figure out where these absorption lines should be.
If I can do it experimentally, that is very useful, because then I have one chart where I know which molecule has which absorption lines that indicate a specific molecule or atom. And those absorption features compared to what I know molecules and atoms will absorb tell me what’s in the chemical makeup of the planet. And then I need to understand what I’m seeing. Does that mean that there’s a geological process going on on that planet? Does that mean that I need to invoke a biosphere to actually make sense of what I’m seeing? Or could this be a world without life or without life’s leaving any signatures I can spot?
And with the James Webb Space Telescope, I can do that for rocky worlds at the right distance. And so we’re doing that right now for a very specific system that’s called the TRAPPIST-1 system. It’s a small red sun, and it has seven Earth-size planets orbiting it. And three of those are in this right distance — not too hot, not too cold.
But, as you were mentioning before, we actually look at all of those planets, because we also want to look for weird or interesting signs of what rocky planets can be like around other stars. And we are taking the data, but we have to wait because we have to add up the data from the planet every time it goes in front of the star because the signal is so small.
LEVIN: So, is it difficult to have the confidence that you actually have a signature of life? How would we really know this wasn’t just part of the process of evolution of a planet?
KALTENEGGER: That’s a great question, and that’s exactly how I started my career in this field, actually. I wanted to know, how can we actually know? If you want to know this, the first thing you do is you look at the Earth. And I said, OK, the Earth has changed so much, it’s our one case where we know how it changed. So how long could we actually spot signs that are not just geological, that you cannot explain just with geology, on our own planet? Just as a first start, right? Because if that doesn’t work on our own planet, then it won’t work anywhere else, because we have the most information here to decipher what’s what.
And so when you look at our own planet, the combination of oxygen with methane, they react to CO2 and water. So if you see oxygen at the same time as you see methane, then you know something’s producing both of these gases. For methane, it could be volcanoes. But for oxygen, if you’re at the right distance — that means not too much water that you could split up to produce oxygen that way, or not too much CO2, which you could also split to produce oxygen that way — then we have no other explanation than, for that oxygen in context with a reducing gas like methane, that is produced by life. And so that works for a planet like ours.
But what about if the geochemistry is completely bizarre? And that’s what we’re working on. We’re trying to envision different kinds of ways this could work, different kind of rocks we could have, different kind of minerals we could have, and what could actually tell us, oh, there’s life, and there’s really not. So that’s where a lot of the theoretical work comes in. Modeling these planets, seeing what the atmosphere would do if I put more of this in, or less volcanoes, or a bigger planet, and figuring out if there is a solid line of evidence that there’s a biosphere needed for these gas combinations.
And so far, we have this oxygen-methane or ozone-methane combination. It depends a little bit which wavelengths you look at, which features you can see. And it has held up, but it needs the context. So you want to know how much CO2 you have to know how much oxygen you can get that way. And you also want to know how much water has been evaporating. So if it’s super hot and steamy on that world, you could also get a lot of oxygen. But by looking at the light, and at the missing light in your spectrum, these absorption features, you could say how much water, how much CO2 there is to figure out the context of this combination of biosignatures.
LEVIN: Now, let’s say you were an alien civilization somewhere in this region. We are looking at them a thousand years ago, let’s say. They’re looking at us a thousand years ago. And what about the Earth’s atmosphere a thousand years ago could they tell that there was life on the planet? And that would’ve of course also been this pre-industrial age, they wouldn’t be seeing the changes that we’re going through right now.
KALTENEGGER: Exactly, that was what made it so interesting to think about our Earth in the context of time. Because when you go back, a thousand years or five thousand years, there would be no technology, no industry. Yes, civilization started to bloom, but they were very localized.
However, the biosphere, so oxygen in our air with the methane, that has started way earlier than you and me. So that started about 2 billion years ago. So since about 2 billion years ago, the gas combination tells you that there’s life on this planet. However, it doesn’t tell you what kind of life. But if you had some technological gases or signatures, then you could actually add that. But that requires even more light, even more time, and even a bigger telescope.
And so currently we’re designing what we call the Habitable World Observatory — that’s the next mission after the James Webb Space Telescope. That is specifically designed for analyzing these rocky worlds. I love the James Webb Space Telescope, but it’s not just an exoplanet telescope. It also is unraveling secrets of black holes, early galaxies. So a dedicated telescope that’s specifically designed to find signs of life in the atmosphere of these small rocky planets is our next step.
LEVIN: Maybe we can imagine what other life forms could be like. I mean, you’re describing star systems that are completely different stars. The suns are not even emitting in the same range of light that we’ve evolved to see. They could be around dead stars. They could be around very blue stars that are completely different spectrum and just be beyond our imagination.
KALTENEGGER: So, I went to Yellowstone, and I saw these beautiful colors, and then I realized that this is different kinds of life on our own planet that I was not aware of, because I was thinking about you and me, and I was thinking about green vegetation. And so, we started here at the Carl Sagan Institute, the Color Catalog of Life, where we take life from every part of the Earth, and we grow it, and we see how it would look in my telescope. Because if you just look for green plants, you’re probably going to not find all the other things that could be life, even on our own planet.
But what could life be like on other worlds? You mentioned the different energy that they would get from their suns — because the color of the suns indicates how much energy each photon has. But, what’s interesting [is] that even on the Earth, we actually have organisms that can thrive under red light. These are generally organisms that live in mats, so the yellow light doesn’t make it all the way down, but red light does. And so we know of organisms even on our own planet that could thrive under different light conditions. But what they could evolve into is a fascinating question, would they also like evolve into dinosaurs and humans or would it look completely different?
Taking the next step and trying to figure out how life could actually look like on another world is an even bigger leap, because the evolution would be different, the conditions would be different, the light would be different. And in that respect, it’s really nice that this combination of oxygen-methane or ozone-methane, if it’s carbon-based life, could be pretty universal. That’s what we’re thinking about. And so what the life really looks like, we won’t be able to tell you. We won’t even be able to tell you if you’re looking at single cellular life, or if you’re looking at a very big alien space dinosaur. But the gas combination should be telling you that there is something out there.
LEVIN: Here on Earth, we know that life begins in a very simple form, and that seems logical, single-celled organisms. It waffled that way for a very long time before it was able to break energetically over the barrier to multicellularity. And then there’s this wonderful and long process of evolution before you have the whole range of life here on Earth. Natural selection, pressure inducing change, and survival of the fittest. Is that what we’re imagining in the rest of the universe as well?
KALTENEGGER: So for now, we do imagine that when oxygen became available that gives you more energy, then the evolution from single cellular to multicellular started, and then even more oxygen made bigger and bigger organisms possible.
Does this work on every planet? It seems logical that it would but without having any other input, that’s where we stand, and then we keep our eyes open and see what we find. When you talk to biologists, they agree that this seems to be the logical approach, but the cautionary tale is that we’re putting this all on a sample of one — our own world, and how it got started.
LEVIN: When is the time in the history of the Earth when you think it would have been most obvious that there were biosignatures in our atmosphere, if you were to look at us from far away?
KALTENEGGER: The first answer I had to that question would be now. But it turns out that when the biggest creatures were wandering the earth, dinosaurs, [the] most oxygen was around. At least, that’s what we can tell from the rock record. And so it turns out that it would actually be easier to spot a Jurassic world than to spot us, just if you’re going for the combination of oxygen with methane.
LEVIN: We think, oh, look at all the evidence in the atmosphere because of the climate crisis that we are impacting the Earth and we’re such a tiny blip in the history of the Earth and it’s hard to know that another civilization would catch us coming and going.
KALTENEGGER: So we are a tiny blip so far, but I am rooting for us. I think we can be a longer blip, but we have to figure out how to actually safeguard our planet and how to mitigate the climate change we’re doing. We figured out we’re doing this, and now we have to save ourselves.
What’s really interesting, as you said, is how are we going to evolve, right? When we’re looking for life we have the information from what it was like on the Earth before, but we have no information on what it’s going to be in the future. Of course, you can bring science fiction in — are we going to go into the direction of being robots or downloading our consciousness? Are we still going to have physical bodies? Are we going to need food and water? And so the different routes that you could go would actually lead to different kinds of biosignatures you could look for. But because they’re hypothetical, the parameter space is so vast of what you could envision.
But when you’re building a mission, a space mission that needs to find life, the way to do it, is to look for what we know life was like until now — so where we have data, where we know how to interpret what we see — and then keep our eyes open for interesting, weird signals, not just for weird geochemistry and weird life, but maybe also for future life that might be way different than what we expect it to be.
LEVIN: So we’re looking for them — alien life — which of course would be the discovery of the century, but is it possible that they’re also looking for us? Can you clarify for people the big difference between the search for extraterrestrial intelligence versus looking for biosignatures in the atmospheres of exoplanets?
KALTENEGGER: It’s a very specific and targeted search for radio signals or other technology signals that could be beamed our way. But if you think about the life of the Earth in a 24-hour clock, the 4.6 billion years so far, then life started around 5 a.m. At noon, the oxygen-methane combination that I talked about comes on. But us, humanity, the last second before midnight, and radio signals just a fraction of that second.
So the question is, what are you trying to find? If you want to find another civilization like ours that you can talk to, you want to concentrate on that fraction of the second in the 24-hour clock. If you want to figure out if there’s life in the universe, you have a longer chance to find it if you have these gas combinations that I was talking about. But of course, it’d be amazing to get a signal and have a message transmitted to us. But one of the things that’s very interesting there too, is to think about would we actually understand the signal? Will we find the message?
And it’s fascinating to think that if there’s life everywhere in the universe, they might have found us, because since 2 billion years you could figure out that there’s life on our planet if they just had our level of technology.
And so one of the questions that I ask is like, where would we be the aliens? Like, who could see us? Because you can figure out which stars have that perfect vantage point that would see the Earth go in front of the sun.
And then, where is everyone? And what I find very funny about that question is our automatic assumption that if anybody were to find us, they would immediately want to talk to us. Because when I do this in class and I ask my students, “I have two planets, one is 5,000 years older than us, one is 5,000 years younger. I have only the resources to go to one of those; which one do you want me to pick?” They reliably pick the more advanced one, because they want to learn something. And so if you turn that around, the question is, “Even if somebody had found us, why would you come to us?” The Earth is my favorite planet. Let me just say that out straight. But we might not be at the adults’ table yet, in terms of what we know.
LEVIN: Now, you’re so enthusiastic about your subject. We like to ask here at “The Joy of Why,” what about your research brings you joy?
KALTENEGGER: When I look up at night, at the sky, and I see those stars, and now I know that there are planets around them. And I know that right now there is an instrument in place that I help point at some of those stars to figure out if we’re alone. I think just that idea, that we’re rewriting our understanding of our place in the cosmos and that I can be part of that, brings me joy. However boring the meeting is, however much admin I have to do, however much my code’s fighting me and not compiling, we are explorers and exploring is always hard.
[Theme plays]
LEVIN: Amazing. We’ve been speaking with astronomer Lisa Kaltenegger about the search for exoplanets in her new book, Alien Earths. Lisa, thanks for joining us.
KALTENEGGER: Thanks so much for having me.
[Theme continues]
LEVIN: Thanks for listening. If you’re enjoying “The Joy of Why” and you’re not already subscribed, hit the subscribe or follow button where you’re listening. You can also leave a review for the show. It helps people find this podcast.
“The Joy of Why” is a podcast from Quanta Magazine, an editorially independent publication supported by the Simons Foundation. Funding decisions by the Simons Foundation have no influence on the selection of topics, guests, or other editorial decisions in this podcast or in Quanta Magazine.
“The Joy of Why” is produced by PRX Productions. The production team is Caitlin Faulds, Livia Brock, Genevieve Sponsler, and Merritt Jacob. The executive producer of PRX Productions is Jocelyn Gonzales. Morgan Church and Edwin Ochoa provided additional assistance.
From Quanta Magazine, John Rennie and Thomas Lin provided editorial guidance, with support from Matt Carlstrom, Samuel Velasco, Arleen Santana and Megan Willcoxon. Samir Patel is Quanta’s editor in chief.
Our theme music is from APM Music. Julian Lin came up with the podcast name. The episode art is by Peter Greenwood, and our logo is by Jackie King and Kristina Armitage. Special thanks to the Columbia Journalism School and Burt Odom Reed at the Cornell Broadcast Studios. I’m your host, Janna Levin. If you have any questions or comments for us, please email us at [email protected]. Thanks for listening.