CRISPR/Cas9 Epigenome Editing Potential for Rare Imprinting Diseases: A Review
- PMID: 32316223
- PMCID: PMC7226972
- DOI: 10.3390/cells9040993
CRISPR/Cas9 Epigenome Editing Potential for Rare Imprinting Diseases: A Review
Abstract
Imprinting diseases (IDs) are rare congenital disorders caused by aberrant dosages of imprinted genes. Rare IDs are comprised by a group of several distinct disorders that share a great deal of homology in terms of genetic etiologies and symptoms. Disruption of genetic or epigenetic mechanisms can cause issues with regulating the expression of imprinted genes, thus leading to disease. Genetic mutations affect the imprinted genes, duplications, deletions, and uniparental disomy (UPD) are reoccurring phenomena causing imprinting diseases. Epigenetic alterations on methylation marks in imprinting control centers (ICRs) also alters the expression patterns and the majority of patients with rare IDs carries intact but either silenced or overexpressed imprinted genes. Canonical CRISPR/Cas9 editing relying on double-stranded DNA break repair has little to offer in terms of therapeutics for rare IDs. Instead CRISPR/Cas9 can be used in a more sophisticated way by targeting the epigenome. Catalytically dead Cas9 (dCas9) tethered with effector enzymes such as DNA de- and methyltransferases and histone code editors in addition to systems such as CRISPRa and CRISPRi have been shown to have high epigenome editing efficiency in eukaryotic cells. This new era of CRISPR epigenome editors could arguably be a game-changer for curing and treating rare IDs by refined activation and silencing of disturbed imprinted gene expression. This review describes major CRISPR-based epigenome editors and points out their potential use in research and therapy of rare imprinting diseases.
Keywords: Angelman syndrome; CRISPR/Cas9; Prader-Willi syndrome; Silver-Russell syndrome; epigenome editing; genomic imprinting; rare disease; transcriptome editing; transient neonatal diabetes mellitus.
Conflict of interest statement
The authors declare no conflict of interest.
Figures
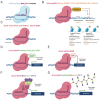
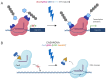
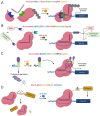
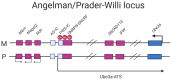
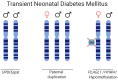
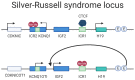
Similar articles
-
Characterization of DNA methylation errors in patients with imprinting disorders conceived by assisted reproduction technologies.Hum Reprod. 2012 Aug;27(8):2541-8. doi: 10.1093/humrep/des197. Epub 2012 Jun 6. Hum Reprod. 2012. PMID: 22674207
-
Prader-Willi Syndrome: Molecular Mechanism and Epigenetic Therapy.Curr Gene Ther. 2020;20(1):36-43. doi: 10.2174/1566523220666200424085336. Curr Gene Ther. 2020. PMID: 32329685 Review.
-
Allele-Specific Epigenome Editing.Methods Mol Biol. 2018;1767:137-146. doi: 10.1007/978-1-4939-7774-1_6. Methods Mol Biol. 2018. PMID: 29524132
-
Association of four imprinting disorders and ART.Clin Epigenetics. 2019 Feb 7;11(1):21. doi: 10.1186/s13148-019-0623-3. Clin Epigenetics. 2019. PMID: 30732658 Free PMC article.
-
Advances in CRISPR-Cas systems for epigenetics.Prog Mol Biol Transl Sci. 2024;208:185-209. doi: 10.1016/bs.pmbts.2024.07.003. Epub 2024 Aug 22. Prog Mol Biol Transl Sci. 2024. PMID: 39266182 Review.
Cited by
-
The Potential of CRISPR/Cas9 Gene Editing as a Treatment Strategy for Inherited Diseases.Front Cell Dev Biol. 2021 Dec 15;9:699597. doi: 10.3389/fcell.2021.699597. eCollection 2021. Front Cell Dev Biol. 2021. PMID: 34977000 Free PMC article. Review.
-
CRISPR-Cas System: The Current and Emerging Translational Landscape.Cells. 2023 Apr 7;12(8):1103. doi: 10.3390/cells12081103. Cells. 2023. PMID: 37190012 Free PMC article. Review.
-
Inadvertent nucleotide sequence alterations during mutagenesis: highlighting the vulnerabilities in mouse transgenic technology.J Genet Eng Biotechnol. 2021 Feb 11;19(1):30. doi: 10.1186/s43141-021-00130-5. J Genet Eng Biotechnol. 2021. PMID: 33570721 Free PMC article.
-
GENE TARGET: A framework for evaluating Mendelian neurodevelopmental disorders for gene therapy.Mol Ther Methods Clin Dev. 2022 Aug 29;27:32-46. doi: 10.1016/j.omtm.2022.08.007. eCollection 2022 Dec 8. Mol Ther Methods Clin Dev. 2022. PMID: 36156879 Free PMC article. Review.
-
Generation and Characterization of a Novel Angelman Syndrome Mouse Model with a Full Deletion of the Ube3a Gene.Cells. 2022 Sep 9;11(18):2815. doi: 10.3390/cells11182815. Cells. 2022. PMID: 36139390 Free PMC article.
References
-
- Kitamoto K., Taketani Y., Fujii W., Inamochi A., Toyono T., Miyai T., Yamagami S., Kuroda M., Usui T., Ouchi Y. Generation of mouse model of TGFBI-R124C corneal dystrophy using CRISPR/Cas9-mediated homology-directed repair. Sci. Rep. 2020;10:1–10. doi: 10.1038/s41598-020-58876-w. - DOI - PMC - PubMed
Publication types
MeSH terms
Supplementary concepts
LinkOut - more resources
Full Text Sources
Other Literature Sources
Medical