Mechanisms and pathologies of human mitochondrial DNA replication and deletion formation
- PMID: 38804971
- PMCID: PMC11346376
- DOI: 10.1042/BCJ20230262
Mechanisms and pathologies of human mitochondrial DNA replication and deletion formation
Abstract
Human mitochondria possess a multi-copy circular genome, mitochondrial DNA (mtDNA), that is essential for cellular energy metabolism. The number of copies of mtDNA per cell, and their integrity, are maintained by nuclear-encoded mtDNA replication and repair machineries. Aberrant mtDNA replication and mtDNA breakage are believed to cause deletions within mtDNA. The genomic location and breakpoint sequences of these deletions show similar patterns across various inherited and acquired diseases, and are also observed during normal ageing, suggesting a common mechanism of deletion formation. However, an ongoing debate over the mechanism by which mtDNA replicates has made it difficult to develop clear and testable models for how mtDNA rearrangements arise and propagate at a molecular and cellular level. These deletions may impair energy metabolism if present in a high proportion of the mtDNA copies within the cell, and can be seen in primary mitochondrial diseases, either in sporadic cases or caused by autosomal variants in nuclear-encoded mtDNA maintenance genes. These mitochondrial diseases have diverse genetic causes and multiple modes of inheritance, and show notoriously broad clinical heterogeneity with complex tissue specificities, which further makes establishing genotype-phenotype relationships challenging. In this review, we aim to cover our current understanding of how the human mitochondrial genome is replicated, the mechanisms by which mtDNA replication and repair can lead to mtDNA instability in the form of large-scale rearrangements, how rearranged mtDNAs subsequently accumulate within cells, and the pathological consequences when this occurs.
Keywords: DNA damage; DNA replication and recombination; mitochondrial dysfunction; mtDNA.
© 2024 The Author(s).
Conflict of interest statement
The authors declare that there are no competing interests associated with the manuscript.
Figures
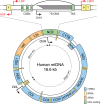
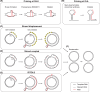
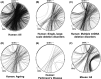
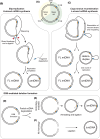
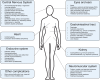
Similar articles
-
Mechanisms of replication and repair in mitochondrial DNA deletion formation.Nucleic Acids Res. 2020 Nov 18;48(20):11244-11258. doi: 10.1093/nar/gkaa804. Nucleic Acids Res. 2020. PMID: 33021629 Free PMC article. Review.
-
Mitochondrial DNA replication and repair defects: Clinical phenotypes and therapeutic interventions.Biochim Biophys Acta Bioenerg. 2022 Jun 1;1863(5):148554. doi: 10.1016/j.bbabio.2022.148554. Epub 2022 Mar 24. Biochim Biophys Acta Bioenerg. 2022. PMID: 35341749 Review.
-
Tissue specific differences in mitochondrial DNA maintenance and expression.Mitochondrion. 2019 Jan;44:85-92. doi: 10.1016/j.mito.2018.01.004. Epub 2018 Jan 12. Mitochondrion. 2019. PMID: 29339192
-
Mitochondrial DNA mutations: an overview of clinical and molecular aspects.Methods Mol Biol. 2012;837:3-15. doi: 10.1007/978-1-61779-504-6_1. Methods Mol Biol. 2012. PMID: 22215537 Review.
-
Replication and Transcription of Human Mitochondrial DNA.Annu Rev Biochem. 2024 Aug;93(1):47-77. doi: 10.1146/annurev-biochem-052621-092014. Epub 2024 Jul 2. Annu Rev Biochem. 2024. PMID: 38594940 Review.
References
Publication types
MeSH terms
Substances
LinkOut - more resources
Full Text Sources
Medical