Heat, Hydration and the Human Brain, Heart and Skeletal Muscles
- PMID: 30671905
- PMCID: PMC6445826
- DOI: 10.1007/s40279-018-1033-y
Heat, Hydration and the Human Brain, Heart and Skeletal Muscles
Abstract
People undertaking prolonged vigorous exercise experience substantial bodily fluid losses due to thermoregulatory sweating. If these fluid losses are not replaced, endurance capacity may be impaired in association with a myriad of alterations in physiological function, including hyperthermia, hyperventilation, cardiovascular strain with reductions in brain, skeletal muscle and skin blood perfusion, greater reliance on muscle glycogen and cellular metabolism, alterations in neural activity and, in some conditions, compromised muscle metabolism and aerobic capacity. The physiological strain accompanying progressive exercise-induced dehydration to a level of ~ 4% of body mass loss can be attenuated or even prevented by: (1) ingesting fluids during exercise, (2) exercising in cold environments, and/or (3) working at intensities that require a small fraction of the overall body functional capacity. The impact of dehydration upon physiological function therefore depends on the functional demand evoked by exercise and environmental stress, as cardiac output, limb blood perfusion and muscle metabolism are stable or increase during small muscle mass exercise or resting conditions, but are impaired during whole-body moderate to intense exercise. Progressive dehydration is also associated with an accelerated drop in perfusion and oxygen supply to the human brain during submaximal and maximal endurance exercise. Yet their consequences on aerobic metabolism are greater in the exercising muscles because of the much smaller functional oxygen extraction reserve. This review describes how dehydration differentially impacts physiological function during exercise requiring low compared to high functional demand, with an emphasis on the responses of the human brain, heart and skeletal muscles.
Conflict of interest statement
José González-Alonso has received funding from the GSSI to undertake fundamental physiological research. The dehydration and hyperthermia studies conducted by José González-Alonso and Steven Trangmar at the Centre for Human Performance, Exercise and Rehabilitation, Brunel University London from 2010 to 2014 were partly supported by a grant from the GSSI.
Figures
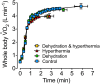
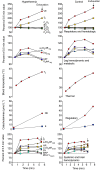
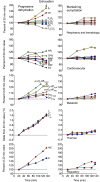
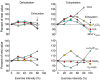
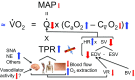
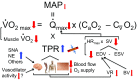
Similar articles
-
Hyperthermia impairs brain, heart and muscle function in exercising humans.Sports Med. 2007;37(4-5):371-3. doi: 10.2165/00007256-200737040-00025. Sports Med. 2007. PMID: 17465611
-
Rehydration strategies--balancing substrate, fluid, and electrolyte provision.Int J Sports Med. 1998 Jun;19 Suppl 2:S133-5. doi: 10.1055/s-2007-971978. Int J Sports Med. 1998. PMID: 9694419
-
Mechanisms of aerobic performance impairment with heat stress and dehydration.J Appl Physiol (1985). 2010 Dec;109(6):1989-95. doi: 10.1152/japplphysiol.00367.2010. Epub 2010 Aug 5. J Appl Physiol (1985). 2010. PMID: 20689090 Review.
-
Metabolic and thermodynamic responses to dehydration-induced reductions in muscle blood flow in exercising humans.J Physiol. 1999 Oct 15;520 Pt 2(Pt 2):577-89. doi: 10.1111/j.1469-7793.1999.00577.x. J Physiol. 1999. PMID: 10523424 Free PMC article.
-
Physiological Function during Exercise and Environmental Stress in Humans-An Integrative View of Body Systems and Homeostasis.Cells. 2022 Jan 24;11(3):383. doi: 10.3390/cells11030383. Cells. 2022. PMID: 35159193 Free PMC article. Review.
Cited by
-
Habitual water intake impacted the body composition of young male athletes in free-living conditions: a cross-sectional study.Front Sports Act Living. 2024 Oct 22;6:1458242. doi: 10.3389/fspor.2024.1458242. eCollection 2024. Front Sports Act Living. 2024. PMID: 39502583 Free PMC article.
-
Lack of sex-specific differences in the associations between the dimensions of great vessels and exercise performance in amateur cyclists.PLoS One. 2024 Nov 4;19(11):e0313165. doi: 10.1371/journal.pone.0313165. eCollection 2024. PLoS One. 2024. PMID: 39495753 Free PMC article.
-
Nourishing Physical Productivity and Performance On a Warming Planet - Challenges and Nutritional Strategies to Mitigate Exertional Heat Stress.Curr Nutr Rep. 2024 Sep;13(3):399-411. doi: 10.1007/s13668-024-00554-8. Epub 2024 Jul 12. Curr Nutr Rep. 2024. PMID: 38995600 Free PMC article. Review.
-
Effects of pre-exercise glycerol supplementation on dehydration, metabolic, kinematic, and thermographic variables in international race walkers.J Int Soc Sports Nutr. 2024 Dec;21(1):2346563. doi: 10.1080/15502783.2024.2346563. Epub 2024 Apr 27. J Int Soc Sports Nutr. 2024. PMID: 38676933 Free PMC article. Clinical Trial.
-
Cognitive Function among Young Women's Football Players in the Summer Heat.Transl Sports Med. 2023 Nov 2;2023:5516439. doi: 10.1155/2023/5516439. eCollection 2023. Transl Sports Med. 2023. PMID: 38654921 Free PMC article.
References
-
- Rowell LB, Brengelmann GL, Murray JA. Cardiovascular responses to sustained high skin temperature in resting man. J Appl Physiol. 1969;27:673–680. - PubMed
-
- Rowell LB. Human cardiovascular adjustments to exercise and thermal-stress. Physiol Rev. 1974;54:75–159. - PubMed
-
- Cheuvront SN, Kenefick RW, Montain SJ, Sawka MN. Mechanisms of aerobic performance impairment with heat stress and dehydration. J Appl Physiol. 2010;109:1989–1995. - PubMed
-
- Sawka MN, Young AJ, Cadarette BS, Levine L, Pandolf KB. Influence of heat stress and acclimation on maximal aerobic power. Eur J Appl Physiol Occup Physiol. 1985;53:294–298. - PubMed
Publication types
MeSH terms
LinkOut - more resources
Full Text Sources
Other Literature Sources
Medical