How glycan metabolism shapes the human gut microbiota
- PMID: 22491358
- PMCID: PMC4005082
- DOI: 10.1038/nrmicro2746
How glycan metabolism shapes the human gut microbiota
Abstract
Symbiotic microorganisms that reside in the human intestine are adept at foraging glycans and polysaccharides, including those in dietary plants (starch, hemicellulose and pectin), animal-derived cartilage and tissue (glycosaminoglycans and N-linked glycans), and host mucus (O-linked glycans). Fluctuations in the abundance of dietary and endogenous glycans, combined with the immense chemical variation among these molecules, create a dynamic and heterogeneous environment in which gut microorganisms proliferate. In this Review, we describe how glycans shape the composition of the gut microbiota over various periods of time, the mechanisms by which individual microorganisms degrade these glycans, and potential opportunities to intentionally influence this ecosystem for better health and nutrition.
Figures
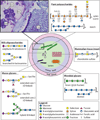
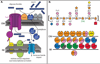
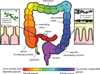
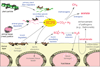
Similar articles
-
Reciprocal Prioritization to Dietary Glycans by Gut Bacteria in a Competitive Environment Promotes Stable Coexistence.mBio. 2017 Oct 10;8(5):e01068-17. doi: 10.1128/mBio.01068-17. mBio. 2017. PMID: 29018117 Free PMC article.
-
Glycan degradation (GlyDeR) analysis predicts mammalian gut microbiota abundance and host diet-specific adaptations.mBio. 2014 Aug 12;5(4):e01526-14. doi: 10.1128/mBio.01526-14. mBio. 2014. PMID: 25118239 Free PMC article.
-
[Fermentative metabolism by the human gut microbiota].Gastroenterol Clin Biol. 2010 Sep;34 Suppl 1:S16-22. doi: 10.1016/S0399-8320(10)70016-6. Gastroenterol Clin Biol. 2010. PMID: 20889000 French.
-
Glycobiome: bacteria and mucus at the epithelial interface.Best Pract Res Clin Gastroenterol. 2013 Feb;27(1):25-38. doi: 10.1016/j.bpg.2013.03.001. Best Pract Res Clin Gastroenterol. 2013. PMID: 23768550 Review.
-
Gut microbiota, host health, and polysaccharides.Biotechnol Adv. 2013 Mar-Apr;31(2):318-37. doi: 10.1016/j.biotechadv.2012.12.009. Epub 2012 Dec 30. Biotechnol Adv. 2013. PMID: 23280014 Review.
Cited by
-
The abundance and variety of carbohydrate-active enzymes in the human gut microbiota.Nat Rev Microbiol. 2013 Jul;11(7):497-504. doi: 10.1038/nrmicro3050. Epub 2013 Jun 10. Nat Rev Microbiol. 2013. PMID: 23748339 Review.
-
Structure and evolution of the bifidobacterial carbohydrate metabolism proteins and enzymes.Biochem Soc Trans. 2021 Apr 30;49(2):563-578. doi: 10.1042/BST20200163. Biochem Soc Trans. 2021. PMID: 33666221 Free PMC article. Review.
-
Some are more equal than others: the role of "keystone" species in the degradation of recalcitrant substrates.Gut Microbes. 2013 May-Jun;4(3):236-40. doi: 10.4161/gmic.23998. Epub 2013 Apr 2. Gut Microbes. 2013. PMID: 23549436 Free PMC article. Review.
-
Structural dissection of a complex Bacteroides ovatus gene locus conferring xyloglucan metabolism in the human gut.Open Biol. 2016 Jul;6(7):160142. doi: 10.1098/rsob.160142. Open Biol. 2016. PMID: 27466444 Free PMC article.
-
Engineering the Microbiome: a Novel Approach to Immunotherapy for Allergic and Immune Diseases.Curr Allergy Asthma Rep. 2015 Jul;15(7):39. doi: 10.1007/s11882-015-0538-9. Curr Allergy Asthma Rep. 2015. PMID: 26143390 Review.
References
-
- Flint HJ, Bayer EA, Rincon MT, Lamed R, White BA. Polysaccharide utilization by gut bacteria: potential for new insights from genomic analysis. Nat Rev Microbiol. 2008;6:121–131. - PubMed
Publication types
MeSH terms
Substances
Grants and funding
LinkOut - more resources
Full Text Sources
Other Literature Sources