On the sequence-directed nature of human gene mutation: the role of genomic architecture and the local DNA sequence environment in mediating gene mutations underlying human inherited disease
- PMID: 21853507
- PMCID: PMC3177966
- DOI: 10.1002/humu.21557
On the sequence-directed nature of human gene mutation: the role of genomic architecture and the local DNA sequence environment in mediating gene mutations underlying human inherited disease
Abstract
Different types of human gene mutation may vary in size, from structural variants (SVs) to single base-pair substitutions, but what they all have in common is that their nature, size and location are often determined either by specific characteristics of the local DNA sequence environment or by higher order features of the genomic architecture. The human genome is now recognized to contain "pervasive architectural flaws" in that certain DNA sequences are inherently mutation prone by virtue of their base composition, sequence repetitivity and/or epigenetic modification. Here, we explore how the nature, location and frequency of different types of mutation causing inherited disease are shaped in large part, and often in remarkably predictable ways, by the local DNA sequence environment. The mutability of a given gene or genomic region may also be influenced indirectly by a variety of noncanonical (non-B) secondary structures whose formation is facilitated by the underlying DNA sequence. Since these non-B DNA structures can interfere with subsequent DNA replication and repair and may serve to increase mutation frequencies in generalized fashion (i.e., both in the context of subtle mutations and SVs), they have the potential to serve as a unifying concept in studies of mutational mechanisms underlying human inherited disease.
© 2011 Wiley-Liss, Inc.
Figures
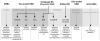
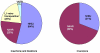

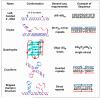
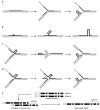
Similar articles
-
Gene deletions causing human genetic disease: mechanisms of mutagenesis and the role of the local DNA sequence environment.Hum Genet. 1991 Mar;86(5):425-41. doi: 10.1007/BF00194629. Hum Genet. 1991. PMID: 2016084
-
The mutational spectrum of single base-pair substitutions causing human genetic disease: patterns and predictions.Hum Genet. 1990 Jun;85(1):55-74. doi: 10.1007/BF00276326. Hum Genet. 1990. PMID: 2192981 Review.
-
Mechanisms of DNA expansion.Chromosoma. 1995 Oct;104(1):2-13. doi: 10.1007/BF00352220. Chromosoma. 1995. PMID: 7587591 Review.
-
Meta-analysis of gross insertions causing human genetic disease: novel mutational mechanisms and the role of replication slippage.Hum Mutat. 2005 Feb;25(2):207-21. doi: 10.1002/humu.20133. Hum Mutat. 2005. PMID: 15643617
-
Alu repeats and human genomic diversity.Nat Rev Genet. 2002 May;3(5):370-9. doi: 10.1038/nrg798. Nat Rev Genet. 2002. PMID: 11988762 Review.
Cited by
-
Long-read sequencing technology indicates genome-wide effects of non-B DNA on polymerization speed and error rate.Genome Res. 2018 Dec;28(12):1767-1778. doi: 10.1101/gr.241257.118. Epub 2018 Nov 6. Genome Res. 2018. PMID: 30401733 Free PMC article.
-
The interaction between cytosine methylation and processes of DNA replication and repair shape the mutational landscape of cancer genomes.Nucleic Acids Res. 2017 Jul 27;45(13):7786-7795. doi: 10.1093/nar/gkx463. Nucleic Acids Res. 2017. PMID: 28531315 Free PMC article.
-
New clinical and molecular insights on Barth syndrome.Orphanet J Rare Dis. 2013 Feb 14;8:27. doi: 10.1186/1750-1172-8-27. Orphanet J Rare Dis. 2013. PMID: 23409742 Free PMC article.
-
Complement component 4 copy number variation and CYP21A2 genotype associations in patients with congenital adrenal hyperplasia due to 21-hydroxylase deficiency.Hum Genet. 2012 Dec;131(12):1889-94. doi: 10.1007/s00439-012-1217-8. Epub 2012 Aug 12. Hum Genet. 2012. PMID: 22886582 Free PMC article.
-
A canine orthologue of the human GFAP c.716G>A (p.Arg239His) variant causes Alexander disease in a Labrador retriever.Eur J Hum Genet. 2016 Jun;24(6):852-6. doi: 10.1038/ejhg.2015.223. Epub 2015 Oct 21. Eur J Hum Genet. 2016. PMID: 26486469 Free PMC article.
References
-
- Abadie V, Lyonnet S, Maurin N, Berthelon M, Caillaud C, Giraud F, Mattei JF, Rey J, Rey F, Munnich A. CpG dinucleotides are mutation hot spots in phenylketonuria. Genomics. 1989;5:936–939. - PubMed
-
- Abeysinghe SS, Chuzhanova N, Krawczak M, Ball EV, Cooper DN. Translocation and gross deletion breakpoints in human inherited disease and cancer. I: Nucleotide composition and recombination-associated motifs. Hum Mutat. 2003;22:229–244. - PubMed
-
- Abo-Dalo B, Kutsche K, Mautner V, Kluwe L. Large intragenic deletions of the NF2 gene: breakpoints and associated phenotypes. Genes Chrom Cancer. 2010;49:171–175. - PubMed
-
- Al-Mahdawi S, Pinto RM, Ismail O, Varshney D, Lymperi S, Sandi C, Trabzuni D, Pook M. The Friedreich ataxia GAA repeat expansion mutation induces comparable epigenetic changes in human and transgenic mouse brain and heart tissues. Hum Mol Genet. 2008;17:735–746. - PubMed
Publication types
MeSH terms
Substances
Grants and funding
LinkOut - more resources
Full Text Sources
Other Literature Sources
Medical